Oilfield and Oilsands Glossary
LINKS TO THE BEST RESOURCES
The Oilfield Glossary: Where the Oil Field Meets the Dictionary [Schlumberger]Oil, Gas and Petroleum Prospecting Term Glossary [MPG Petroleum Inc.]
Frequently Asked Questions (FAQs) Concerning Federally-Regulated Petroleum Pipelines in Canada [National Resource Canada; Government of Canada]
Canadian Energy Pipeline Association - Resources
The Engineering Science of Oil Pipelines [Science Media Centre of Canada]
QUICK VIDEO - How we make oil by Syncrude
ARTICLES
Oil Sands Magazine
OIL SANDS 101: PROCESS OVERVIEW
OIL SANDS GEOLOGY & THE PROPERTIES OF BITUMEN
IN-SITU BITUMEN EXTRACTION // Up-to-date Data/Maps - In-Situ
BITUMEN UPGRADING EXPLAINED // Up-to-date Data/Maps - Upgraders
OIL SANDS 101: PROCESS OVERVIEW
PROCESS OVERVIEW
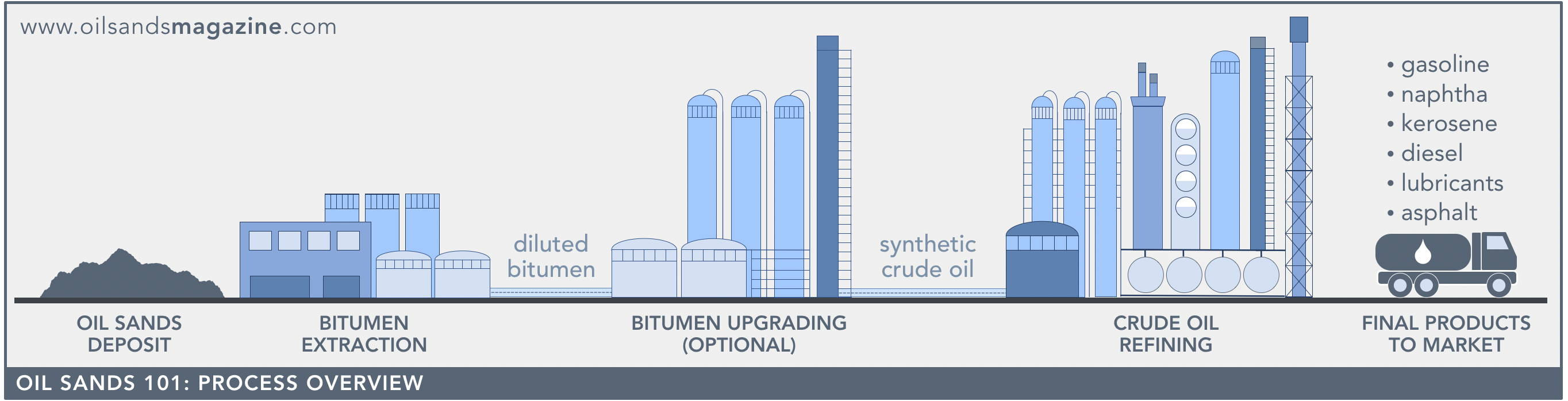
IN-SITU BITUMEN EXTRACTION
About 80% of Alberta's bitumen reserves are too deep to be mined and must be extracted in-place (or in-situ) using steam. The bitumen is heated and pumped out of the ground, leaving most of the solids behind. In-situ extraction has a much smaller footprint than oil sands mining, uses less water and does not produce a tailings stream.
TYPICAL FLOWSHEET
All commercial in-situ facilities contain four basic components:
BITUMEN PRODUCED IN-SITU • CLICK FOR LIVE CHART →
THE IN-SITU ADVANTAGE
1. Cyclic Steam Stimulation (CSS)
2. Steam-Assisted Gravity Drainage (SAGD)
Both CSS and SAGD have identical central processing facilities. The difference lies in the positioning and number of wellheads.
1. CYCLIC STEAM STIMULATION (CSS)

Imperial Oil's Cold Lake facility was the first in Canada to commercialize CSS recovery of bitumen. Initial recovery rates were only 20-25%, but recent improvements in technology have boosted recovery rates into the 50% range for some operations.
RECOVERY RATES AT COLD LAKE (COURTESY IMPERIAL OIL)
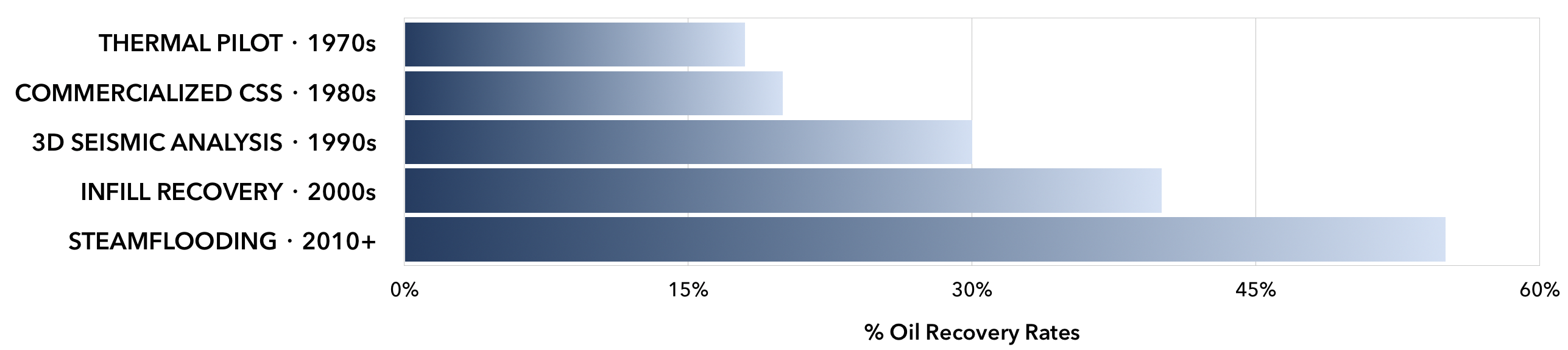
2. STEAM-ASSISTED GRAVITY DRAINAGE (SAGD)

CSS VS SAGD: Note that the choice between CSS and SAGD technology is dictated by the geology of the reservoir. The Cold Lake deposit lends itself better to CSS while oil sands in the Athabasca region responds better to SAGD.
Here's a list of the largest in-situ facilities currently in operation in the oil sands:
[GOOGLE MAP - IMAGE @ BOTTOM plus TABLE]
BITUMEN UPGRADING EXPLAINED
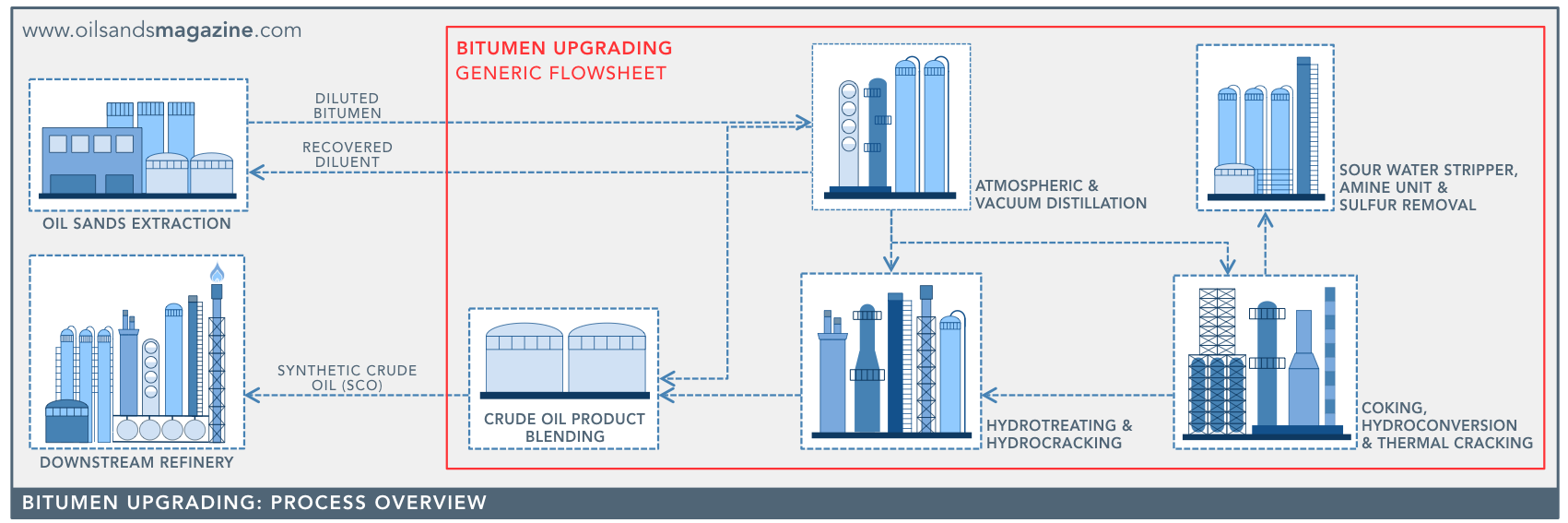
Part 2: Building Crude Oil Pipelines: How to Make Preliminary Cost Estimates
Published by: Rick Smead
In Part 1 of this series we discussed the fact that new pipeline development is driven by either need or opportunity, and more often than not, a combination of the two. The key question that pipeline developers and their customers (the shippers) have to consider before committing to build new capacity, we said, is whether it will “pay” to flow crude on the pipeline once it’s built––not just the first year or the first three, but for years if not decades to come. To answer this question, pipeline developers and shippers have to consider both current and future economics. There are three fundamental factors that drive pipeline economics: 1) future supply dynamics (and the resulting price impact) at the origination point (Point A); 2) future demand (and price) at the destination point (Point B); and 3) the transportation cost to flow crude from Point A to Point B. In Part 2, we focused on estimating capital costs. We went through a geometry exercise to confirm an already-popular industry rule-of-thumb for estimating the diameter of the pipe to reach a certain capacity. We also explained and used a “cost per inch-mile” approximation to figure out what the pipe itself would probably cost, then included standard rough estimates of the cost for everything else: the pumps, storage, and meters necessary for the pipeline to work. We confirmed those numbers by reviewing some pending new projects, and ultimately got to the example of a 200-Mb/d crude oil pipeline 500 miles long costing $990 million to build.
Today, we get to the heart of the matter: What per-barrel rate needs to be charged for a pipeline project to be worthwhile to the developer? And––just as important––would the rate be low enough to attract sufficient commitments from crude oil shippers? One preliminary warning: We noted that this series on oil pipeline economics is similar in a lot of ways to our But I Would Pipe 500 Miles series on the economics of natural gas pipelines. As in that effort, our aim is to present simple, rule-of-thumb approaches to determining whether an oil pipeline project might be worth pursuing, not to undertake the detailed engineering analysis that would be needed to really develop one. So ours is the calculation that tells you whether to think more about a prospective project, not the calculation that would get someone to give you $990 million to build a 200-Mb/d, 500-mile pipeline—that takes a bunch of engineers and estimators.
Now to the task of estimating our per-barrel rate. In addition to knowing the capacity and capital cost of the pipeline, we need to know a couple of other important things to be able to establish what the pipeline’s rate would be. First, we need to know the operating costs––the annual out-of-pocket cost of actually running the pipeline (sometimes including having to heat the pipeline––or add diluent or lubricant––to keep heavy oil flowing). Second, we need to recognize that the pipeline won’t always run at full capacity. If it will charge by the barrel that actually flows, we need to estimate the pipeline’s “load factor,” or the average flow divided by the capacity.
Since we’ve now been in “rule-of-thumb” country for a while, that would be the best place to look for these answers as well. A typical developer would want to work out representative numbers to fill in all those operating-cost blanks: the staffing of the pipeline; the average salary and benefits for employees; the maintenance cost per mile; property and any other local taxes; the variable operating costs (such as pump electricity) that change with actual throughput; and the administrative overhead that would have to be covered. (As we indicated, there might be additional costs for a heavy-crude pipeline: to heat the stuff, and or to add diluent or special lubricants. These heavy-crude issues are so case-specific that we won’t clutter up our rule-of-thumb with them.) Even without the complication of enabling heavy crude to move through the pipe, it’s still a lot of factors to consider. To streamline the process, we’ve reviewed the results of the estimates of some of our favorite pipeline developers and made two significant conclusions: 1) fixed operating costs have a reliably consistent relationship with a pipeline’s capital cost (which, of course, is largely a function of the project’s length and capacity); and 2) the variable operating costs generally fall within a narrow cents-per-barrel range.
One more thing about operating costs––detail doesn’t matter much, because for any new pipeline, the impact of operating costs on the total annual revenue requirement is always a lot less than the impact of recovering the capital cost of the pipeline itself at a decent internal rate of return (IRR). In other words, don’t sweat the small stuff when the rule-making thumb is at work. Taking all of this into account and breaking out variable cost separately, the “first-look” quick estimate of the annual fixed operating costs that will need to be charged can reasonably be expected to be between 6 and 7% of the original capital cost—let’s call it 6.5%. And that variable cost we broke out separately is typically 30 cents per barrel, if the thick-crude issues we mentioned before aren’t taken into account.
Now, we take on those capital cost recovery and IRR issues. To do that, we need to determine the period of time over which we would like to recover our capital costs, as well as establish a reasonable IRR. For crude oil pipelines, the capital cost recovery period typically ranges from 10 to 20 years––it all depends on how strong the developer believes the market will be, or the type and length of contracts that are expected to be signed. In the case of the kind of large-capacity, long-distance pipeline we use as an example here, we’re going to assume that no one would go through the trouble of building the project if the business case for it were going to last less than 20 years. Meanwhile, as we’ll discuss in the next installment of this series, regulators will usually force capital cost recovery periods that are even longer––as long as 35 years. So let’s use 20 years for our rate wish-list. For a cost of money, the most typical IRR we’ve seen is 10%. Is this before taxes or after taxes? Yes. That is, there’s not another adjustment for income tax costs, but in a lot of cases pipeline developers are able to sort all of that out later, whether through the way their companies are structured—either as flow-through entities such as master limited partnerships (MLPs – see Masters of the Midstream), or as high-leverage (high-debt) investors with a lot of tax deductions. In other words, don’t worry about income taxes here, we’ll address them again in our regulatory explanation.
The end result is that we need an annual revenue level that will reimburse all the operating costs, pay off the original investment over 20 years, and earn a 10% IRR. That can just be a constant annual revenue level, designed like a house payment plus expenses. So we run a payment formula with a “rate” of 10%, a “period” of 20 years, and a “present value” of the original capital cost—in this case, $990 million. Then we add 6.5% of that capital cost to the answer for fixed operating expenses, to arrive at the dollars the pipeline needs to charge in a year to achieve its 10% IRR.
Using the Excel “payment” function, the capital-related annual payment looks like this:
= PMT (10%, 20, $990,000,000) = $116,285,029
Adding in fixed operating expenses at 6.5% of the capital investment of $990 million, the total revenue target for fixed costs looks like this:
Operating Expense = 6.5% X $990,000,000 = $64,350,000
+ $116,285,029 for Capital Recovery and Time Value
= $180,635,029 Total Revenue Target (round to $181 million)
The variable cost, already stated as 30 cents per barrel will be added at the end. So for the last step, turning all of this into a rate, it goes like this: At 200 Mb/d, full (100%) use of the pipeline would mean it would move 73 million barrels a year (200 Mb/d x 365 days). So if the shippers were expected to use the pipeline all the time at full capacity––or if the pipeline had agreements where they pay the rate as if they’re doing so––the rate would be:
$181 million divided by 73 million barrels, or $2.47, plus $0.30 variable cost = $2.77 per barrel.
But if the shippers will just pay as they go, based on actual use, we have to estimate how often they’ll really use the pipeline. A typical assumption is that they’ll use it at 60% percent of capacity, or a 60% load factor. So now, the annual volume is 73 million barrels times 60%, or 44 million barrels. So now the rate would look like this:
$181 million divided by 44 million barrels, or $4.11, plus $0.30 variable cost = $4.41 per barrel.
So depending on the market conditions and contract structures you assume, that would be the range$2.77 to $4.41 per barrel––to make the developer whole for the investment and out-of-pocket expenses over a 20-year life.
A final––and critically important––consideration is whether the pipeline project’s rates need regulatory approval. If the pipeline crosses state lines and ships for any third parties, it would be regulated by the Federal Energy Regulatory Commission (FERC). If the pipeline would be entirely within one state’s boundaries, the degree to which its rates would be regulated (if at all) vary widely, from “let’s make a deal” laissez faire to being regulated like a public utility. And for each regulator, whether FERC or at the state level, the history and methods of rate regulation are complicated and multi-faceted. So that comes next: Will the pipeline be able to charge its $2.77 to $4.41 per barrel for life, after the regulator gets done with it? Stay tuned.
In Part 1 of this series we discussed the fact that new pipeline development is driven by either need or opportunity, and more often than not, a combination of the two. The key question that pipeline developers and their customers (the shippers) have to consider before committing to build new capacity, we said, is whether it will “pay” to flow crude on the pipeline once it’s built––not just the first year or the first three, but for years if not decades to come. To answer this question, pipeline developers and shippers have to consider both current and future economics. There are three fundamental factors that drive pipeline economics: 1) future supply dynamics (and the resulting price impact) at the origination point (Point A); 2) future demand (and price) at the destination point (Point B); and 3) the transportation cost to flow crude from Point A to Point B.
In Part 2, we focused on estimating capital costs. First, we went through a geometry exercise to confirm an already-popular industry rule-of-thumb for estimating the diameter of the pipe to reach a certain capacity. Then we explained and used a “cost per inch-mile” approximation to figure out what the pipe itself would probably cost, inclusive of all the other stuff—pumps, storage, and meters—that go with the pipe. We confirmed the numbers based on some real-world projects, and ultimately estimated that our hypothetical 200 Mb/d pipeline, 500 miles long, would cost $990 million. The next step, in Part 3, was to figure out how much the developer would need to charge to pay off that $990 million pipeline and earn a decent internal rate of return, or IRR. The answer was a range of $2.77 to $4.41 per barrel, depending on how much oil moved and how the contracts were structured.
But now, we come to a crucial question: Will the developer be able to charge that much, if a Federal or state regulator gets to review the rates? What does it mean for the pipeline to be regulated? For the pipeline developer and owner, it can be a bunch of new hoops to jump through and keep jumping through, depending on who does the regulating. For the shipper, it can be a whole bunch of extra tools to get bargaining leverage with the pipeline, or even just to force the rates down over the life of a contract. But meanwhile, if regulation is too unfriendly, too likely to deny the pipeline owner the ability to make enough money, it can keep anyone from wanting to build the pipeline in the first place.
The impact and mechanics of rate regulation depend a lot on who does the regulating. We’ll focus most heavily on Federal regulation, since it’s the most complicated. Very simply, interstate pipelines, that actually cross one or more state lines, get regulated by the Feds. Which Feds? The Federal Energy Regulatory Commission, or FERC, the same agency that regulates natural gas pipelines. But that is where any similarity ends. FERC regulation of oil pipelines is based on the Interstate Commerce Act, the same law that governs trucks and other forms of interstate transportation, whereas natural gas pipelines are regulated according to the Natural Gas Act—a law crafted just for them. Up until 1977, oil pipelines were regulated by the Interstate Commerce Commission (ICC), which had its own pretty simple ways of doing things. The ICC let a pipeline set rates to recover its out-of-pocket costs, pay for depreciating the pipe, and earn a return on a “valuation” rate base.” We don’t need to get into that here, since it’s long-gone.
In 1977, through the Energy Organization Act, the Federal Power Commission was turned into the FERC, the Department of Energy was created, etc. Regulation of oil pipelines was moved from the ICC to the FERC, which also had its own way of doing things. The next couple of decades saw various changes by FERC and some incredibly complicated (and boring) ways of dealing with the transition. Since today the rules are pretty well settled, and especially for a brand-new pipeline none of that tortuous history matters much, we will keep it to ourselves. Besides, we never want to put you to sleep on your keyboard only to find a bunch of G’s in the middle of an email thanks to nose typing.
We will, however, arm you to be able to sound knowledgeable if you’re ever at an oil-pipeline cocktail party. Just remember:
Opinion 154B, in 1985: “Wasn’t 154B when FERC really took hold and pushed its own way of doing things on oil pipelines?”
Energy Policy Act of 1992: “Good thing Congress streamlined things in EPAct92 and let FERC give us some consistent rules. Wow, ‘Congress’ and ‘streamlined’ in the same sentence—I must be dreaming.” And
Order No. 561 in 1992: “Yeah, when they really made indexing the primary way to set rates, it sure saved a lot of uncertainty and lawyer costs.”
Then, if anyone presses you, say, “That’s all ancient history” and count on any further discussion to lose the audience anyway. If you want to know much more detail, go to an Association of Oil Pipelines meeting sometime and get the full story.
But now back to the present: When an interstate oil pipeline starts shipping oil for third parties—whether it’s a new pipeline or an existing pipeline that previously just shipped its owner’s oil, it has to file a tariff laying out rates that will apply equally to each type of customer. Those rates are capped at either a rate agreed upon by a shipper not affiliated with the pipeline, or by a cost-of-service study. There are other options for setting rates once the pipeline is in service—most notably “market-based” rates, if the pipeline can prove lack of market power, where there are a ton of other issues. But we won’t get into them here, we’re just trying to decide whether to build the thing in the first place.
What’s a cost-of-service study? It’s the same thing any regulated utility (or gas pipeline, for that matter) does in a rate case. The rates are based on the cost of doing business:
Then the total cost of service gets divided by the expected billed throughput. This can be where we get into two different kinds of shippers: “Committed” and “Uncommitted.” Frequently shippers who have committed to long-term contracts get billed as if they took their full contract every day.
Uncommitted tend to be the rest of the industry, paying as they go. So, for example, if a pipeline were half committed and half uncommitted, and the uncommitted shippers were expected to fill 60 percent of the uncommitted capacity, the “billed throughput” would be the full committed contracts at 100 percent, and uncommitted at 60 percent, for an overall load-factor, or billing level, of 80 percent of the total capacity.
So let’s run through an example of FERC’s rate setting methodology, based on the capital cost, operating cost, and range of throughput we worked out for the pipeline developer’s economic target in Part 3 of this blog series:
Variable Operating Cost: 30 cents per barrel
Fixed Cost of Service
Fixed Operating Cost: $64,350,000 (this is from Part 3
Depreciation: $33,000,000 (Capital cost of $990 million over a typical FERC 30-year life)
Return on Investment: $74,250,000 (7.5% return, based on a 10% return on equity, less 1% inflation, and interest on debt of 6%, equity and debt weighted at 50% each of the capitalization—but don’t sweat it, it’s reasonable)
Income Taxes: $37,867,500 (tax on the return on equity piece, plus tax on the tax)
Adjust for FERC Rules: ($1,485,000) (FERC requires a reduction in the investment being earned on, to reflect half a year’s depreciation and deferred income taxes—don’t ask—which is practically worth only 15 hundredths of a percent of the capital cost)
Fixed Cost of Service: $207,982,500 (Note that all of these items are based on a percentage of the capital cost—even fixed operating cost, which was estimated at 6.5 percent of capital cost back in Part 3. So if you add up the percentages, it comes out to about 21 percent—so you can just cut this whole thing short by multiplying the capital cost by 21 percent—we just wanted to show you why, before we gave you the quick way.)
Using the 200,000 barrel per day capacity and the 80 percent average load factor we explained above, the total billed throughput in a year would be 200,000 X 80% X 365 days, or 58,400,000 barrels per year.
Dividing the fixed cost of service by that, and adding back the 30 cents per barrel variable cost, the rate would be $3.86 per barrel.
Remember, the range of rates we got in Part 3, to come up with what would probably make a developer happy? It was $2.77 at 100 percent load factor, to $4.41, at a 60 percent load factor. The average of those two, which would be the answer at the 80 percent load factor we’re assuming here, would be $3.59 per barrel. So the bottom line to all of this agony is that, at least initially, typical FERC ceiling rates would actually be higher than what the developer needed to achieve a 10 percent internal rate of return over ten years.
So far so good. But the developer was counting on a constant rate over ten years, not one that trails off over time, the way cost-of-service rates do. So what happens next?
What happens next is indexing. Under FERC’s rules, once the initial ceiling rates are set, the pipeline gets to or has to adjust them according to inflation based indices FERC publishes once a year (see Figure 2). This keeps the regulated rate cap not only flat, as the Part 3 formulation did, it lets it increase, although from time to time it makes it decrease a little.
Figure 2 - FERC Oil Pipeline Index
(Per FERC Regs at 18CFR342.3)
Over the last ten years, these annual index postings have allowed oil pipeline rates to go up by a combined 44 percent, meaning that the rate level the developer would need, starting 27 cents below the cap and getting more headroom every time the index goes up, should be very safe from being capped by a regulated rate.
But wait—naturally, there are still a couple of flies in the ointment for the developer. First, as with everything else we’ve done in this series, our regulated ceiling estimate is based on typical results, “rules of thumb,” and in any individual case every piece of the cost of service and throughput denominator might get beaten up by FERC staff and intervenors if they decide to make an issue of it. Second, there is always the looming risk that FERC will call in the developer to true up to a new cost of service later. Oil pipelines have to report their approximate cost of service every year in FERC Form 6, on “page 700” (page 700? Yes, regulation comes with a lot of paper). If the cost of service is a lot lower than the indexed rate, FERC can reduce the ceiling. And third, the rules can change. Remember that “income tax” allowance, about 18 percent of the fixed cost of service? Twice, as recently as this July, the US court of appeals has told FERC to go back to the drawing board in allowing it, since most oil pipelines are part of master limited partnerships (MLPs) that don’t pay income taxes themselves—they flow the liability through to their owners-partners. So the industry has to keep fighting to maintain that major chunk of cost of service in its rate ceilings, and the risk of losing it is always there.
For crude oil pipelines, it’s often not enough to get along with their shippers and keep FERC activity from going on. As we indicated back in Part 1, the same rules apply to refined-product pipelines, who have customers such as airlines, and thus usually end up in a lot more litigation. When those pipelines lose a big policy decision (like the tax allowance), it applies to everyone. So regulation does always include some risk. For the shipper, though, regulation means that there is always someplace to go if the shipper is unhappy with the rates.
So that’s our whirlwind tour of Federal rate regulation. Pipelines that never get outside of one state are regulated by state agencies (like the Texas Railroad Commission-TRRC, or the California Public Utilities Commission-CPUC). We mention those two because they’re the extremes of how states regulate crude oil pipelines. In Texas, unless shippers complain, the pipeline can usually make its deals with shippers and report the numbers to TRRC. (We are in the middle of a blog series on Texas intrastate pipelines that goes into a lot more detail on this. See Floodin' Down in Texas.) But in California, crude oil pipelines are regulated as utilities, just like the local gas or electric company, with very strict, rigid, and low cost-of-service standards. So it’s much harder to make a decent return on a crude-oil pipeline in California than it is in Texas. Other states fall in broad ranges in-between the two.
Wrapping up our series, we hope we’ve given you enough to estimate when and how a crude-oil pipeline can make economic sense, and whether to worry much about regulation. The other pieces of the puzzle—will there be enough oil long-term to support the pipeline, will it be more economic over time than rail, and will the price basis differential hold up for the life of the pipeline—take a lot of review of all the work RBN does around crude oil markets in the United States. So for now, our rule-making thumb will rest.
New Crude and Diluent Pipelines and Storage Capacity in Alberta
Several oil-sands expansion projects committed to when crude oil prices were double what they are today are finally coming online, and midstream companies active in Alberta are building new crude/diluent pipelines and storage capacity to keep pace. New storage caverns for natural gas liquids are also in the works, giving a much-needed boost to Canada’s Energy Province. Today we conclude our series on midstream infrastructure under development in or near Western Canada’s oil sands region that move and store hydrocarbon liquids.
Alberta––sometimes called “the Texas of the North”––has experienced as many ups and downs in its oil patch as the Lone Star State, and lately, with crude prices hovering near $50/bbl and the Fort McMurray, AB area still rebuilding from the wildfires of May 2016 (see Back in the High Life and Over the Hills and Far Away), it’s fair to say that times have been better. The good news is that oil prices have been inching up; a number of the projects committed to a few years ago to boost oil sands production are approaching commercial operation; and the expected output increases are spurring the development of new midstream infrastructure. In Part 1 of this series, we discussed the challenges that oil sands producers have faced (low crude prices, the wildfires, the extra costs associated with moving bitumen and heavy oil to market, etc.), as well as the fact that Alberta producers decided a few years ago (when Western Canadian Select – WCS - crude prices topped $70 to $90/bbl) to undertake a combined 850 Mb/d or so of oil sands capacity-expansion projects that would come online in the 2015-19 period. Those projects have had a domino effect, resulting in the need for a lot more diluent––either in the form of natural gasoline (a natural gas liquid, or NGL) or field condensate (superlight crude oil)––to decrease the viscosity of bitumen and heavy oil and enable them to flow through pipelines. In Part 2, we discussed how the need for more diluent has encouraged natural gas producers in Alberta to focus on “wet” gas and field condensate production in the province’s Montney and Duvernay plays, and how that shift has spurred the development of new natural gas processing capacity (to extract NGLs and remove impurities from raw gas) and fractionation capacity (to separate mixed NGLs into purity products like ethane, propane, butane and natural gasoline––the leading diluent material). Taken together and if they all happen, the Alberta projects we discussed would add 163 Mb/d of fractionation capacity and 800 MMcf/d of natural gas processing capacity; projects just over the border in British Columbia would add another 1 Bcf/d of gas processing capacity.
This time it’s all about new pipelines and storage being built within Alberta’s borders. The aims of these projects (like a lot of midstream work) are a combination of catch-up and in-anticipation-of––that is, the new infrastructure will address current shortfalls in pipeline and storage capacity and provide the incremental midstream capacity that the new oil sands (and other) production will require when the expansion projects we discussed in parts 1 and 2 come online. We begin with Alberta pipeline projects, the longest of which is the 286-mile Grand Rapids Pipeline (blue dashed line in Figure 1), which will actually consist of two parallel pipelines flowing in opposite directions between Edmonton, AB and an oil sands production area northwest of Fort McMurray.
Figure 1: Planned Alberta crude and diluent pipelines; Sources: RBN and company presentations
A 20-inch-diameter pipeline will transport up to 225 Mb/d of diluent north from Edmonton to the oil sands area near Fort McMurray, and a 36-inch-diameter pipeline will move up to 900 Mb/d of diluted bitumen or “dilbit”––a blend of diluent and bitumen (see Heat It for more on dilbit) from the Fort McMurray/oil sands area to Fort Saskatchewan (a half-hour’s drive northeast of Edmonton) for now and to Edmonton when market conditions warrant. All but the Fort Saskatchewan-to-Edmonton crude pipeline section will become operational in 2017. A 50-50 joint venture (JV) of TransCanada Corp. and Brion Energy owns most of the Grand Rapids Pipeline project; in August 15 the JV agreed to sell a half-stake in the 28-mile Edmonton-to-Fort Saskatchewan section of Grand Rapids’ northbound diluent pipeline to Keyera Corp., whose Edmonton terminal is the pipeline’s starting point.
The next-longest pipeline under construction in Alberta is the 269-mile Norlite Pipeline (purple line in Figure 1), a 70/30 JV of Enbridge and Keyera that will transport up to 218 Mb/d of diluent from Enbridge’s Stonefell terminal near Fort Saskatchewan to Enbridge’s Fort McMurray South terminal (which is located just where its name suggests), with a transfer line to Suncor’s East Tank Farm. The 24-inch-diameter Norlite project (also known as Line 74), whose capacity is expandable to 465 Mb/d, will come online in the first half of next year (2017). Enbridge, which in September 2016 unveiled a plan to merge with Spectra Energy, is planning a pair of 36-inch-diameter pipeline projects that together will move dilbit south from its Cheecham, AB terminal to Hardisty, AB. The first is the Wood Buffalo Extension (green line), which would––wait for it––extend Enbridge’s existing Wood Buffalo Pipeline (which runs from the company’s Athabasca Terminal near Fort McMurray to Cheecham, and which is also known as Line 75). The 65-mile extension (south to Kirby Lake, AB) will carry up to 800 Mb/d of dilbit when it starts up next year. The second 36-inch-diameter project is a “twinning” of the 214-mile portion of Enbridge’s Athabasca Pipeline (aka Line 19) from Kirby Lake to Hardisty. The Athabasca Twin Pipeline (orange line) will add 800 Mb/d of southbound dilbit capacity and is planned to begin operating by mid-2017. By the way, the combo of the Wood Buffalo Extension and the Athabasca Twin Pipeline will be known as Line 45.)
That’s a lot of new crude and diluent pipeline capacity, and it’s matched by a slew of planned Alberta storage capacity, not just for crude and diluent but for mixed NGLs, ethane and other purity NGLs.
They include:
As we said, these crude/diluent pipelines and crude/diluent/NGL storage facilities are being developed both to meet existing needs and to prepare the market for the new oil sands production capacity that will be coming online. Whether this round of Alberta’s midstream projects will be the last for a while will depend to some degree on whether we’ve seen the last of the big oil sands expansions. Producers have been working to improve efficiencies and reduce costs, but further investment in bitumen production can be a tough sell with crude prices at current levels.
OIL SANDS GEOLOGY & THE PROPERTIES OF BITUMEN
IN-SITU BITUMEN EXTRACTION // Up-to-date Data/Maps - In-Situ
BITUMEN UPGRADING EXPLAINED // Up-to-date Data/Maps - Upgraders
CANADIAN REFINERIES // includes Up-to-date Data/Maps
CRUDE OIL and DILUENT TERMINALS // includes maps
CRUDE OIL and DILUENT TERMINALS // includes maps
RBN Energy LLC
OIL SANDS 101: PROCESS OVERVIEW
PROCESS OVERVIEW
Petroleum products are produced from the oil sands through 3 basic steps:
- Extraction of the bitumen from the oil sands, where the solids and water are removed
- Upgrading of the heavy bitumen to a lighter, intermediate crude oil product
- Refining of the crude oil into final products such as gasoline, lubricants and diluents.
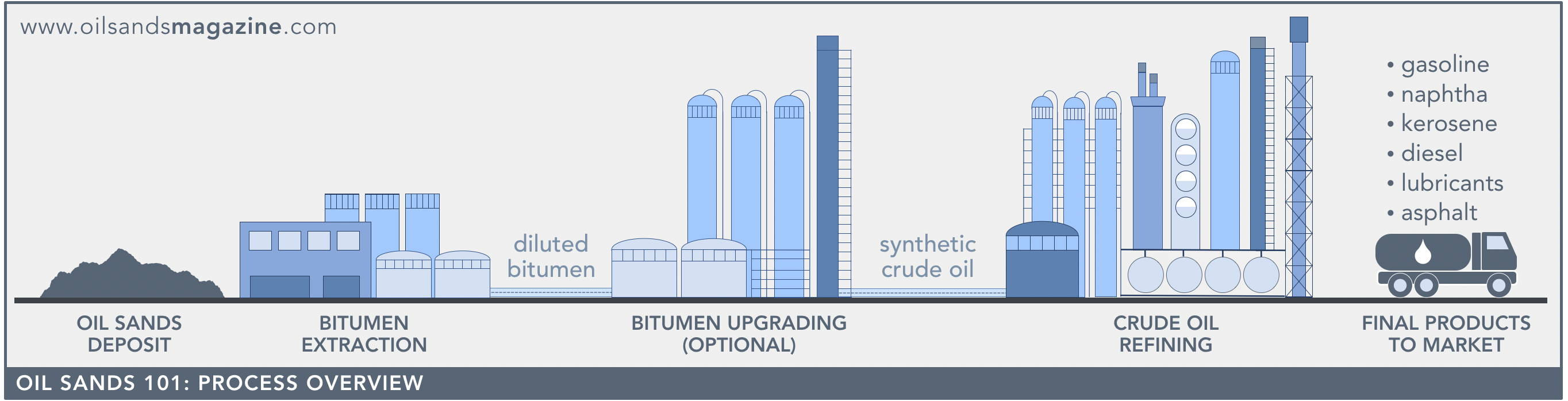
IN-SITU BITUMEN EXTRACTION
About 80% of Alberta's bitumen reserves are too deep to be mined and must be extracted in-place (or in-situ) using steam. The bitumen is heated and pumped out of the ground, leaving most of the solids behind. In-situ extraction has a much smaller footprint than oil sands mining, uses less water and does not produce a tailings stream.
Only 20% of Alberta's bitumen reserves are close enough to the surface to be mined. The remainder can only be feasibly extracted in-situ (or in place) using steam. Although in-situ bitumen extraction traditionally had very low recovery rates, recent advancements in technology have allowed for significant process improvements. Smaller processing plants and higher production rates have greatly improved the profitability of in-situ extraction, which has become increasingly more popular in the oil sands and expects to lead the growth in bitumen production through the next few decades.
In-situ facilities consist of the following basic unit operations:
In-situ facilities consist of the following basic unit operations:
- a series of well pads scattered throughout the oil sands deposit
- a steam and power generation plant which provides power for the facility and high-pressure steam for injection into the wellheads
- a central processing plant where the oil and water emulsion produced at the injection wells is separated
- a water treatment plant where the recovered water is cleaned and recycled back into the process, and
- a product storage facility where the bitumen is diluted with condensate for storage and transportation via pipeline.
TYPICAL FLOWSHEET
All commercial in-situ facilities contain four basic components:
- well pads
- steam generation
- bitumen production, and
- water treatment.
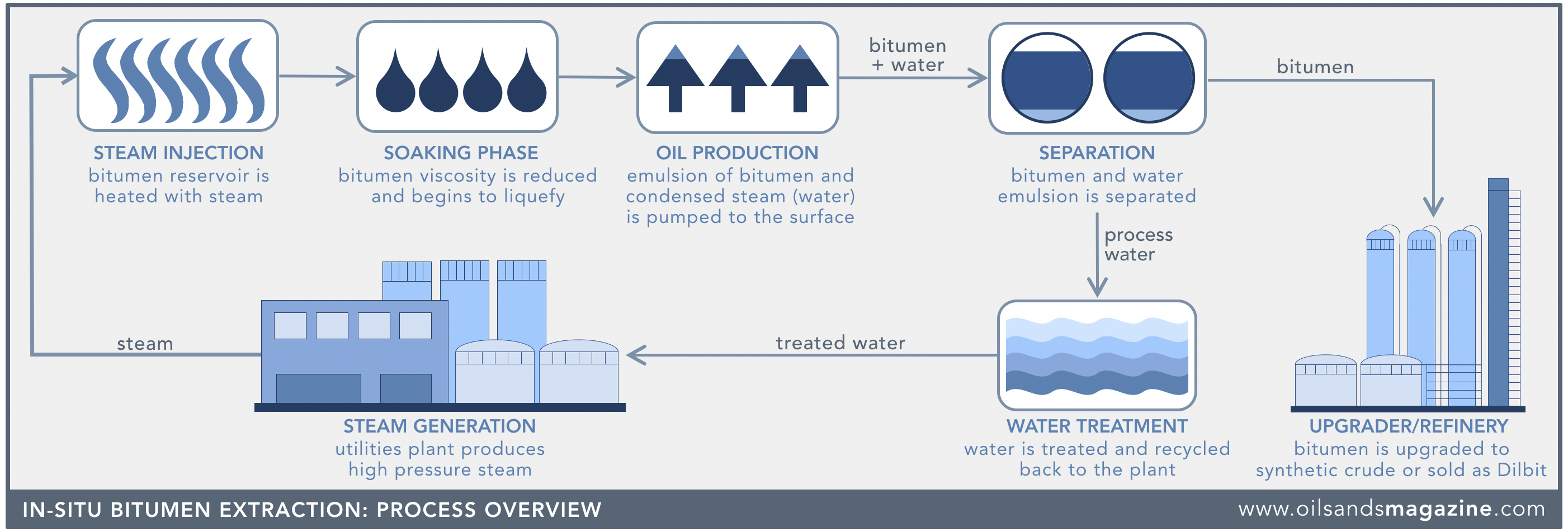
IN-SITU VS MINING: PROS AND CONS
In-situ extraction of bitumen has become increasingly popular in recent years and expects to lead the growth in bitumen production over the coming decades. In-situ facilities can be a lot smaller and do not require the same economy of scale as oil sands mining operations, making them cheaper and faster to bring online.
BITUMEN PRODUCED IN-SITU • CLICK FOR LIVE CHART →
THE IN-SITU ADVANTAGE
- Smaller footprint: The surface area required for the wellheads is very small relative to the oil sands reserve deposit size. In-situ facilities therefore have a much smaller footprint than surface mining operations. In-situ requires about 1/7th the land area of an equivalent-sized mining facilities. Alberta's oil sand mining footprint is actually only 3% when measured by square footage.
- More efficient water usage: In-situ operations use water for steam production, which is mostly recovered and recycled back into the process plant. Fresh water consumption rates are therefore much lower than mining operations which require large volumes of water to slurry the oil sands. In-situ facilities typically require 1/5th the fresh water volume of an equivalent-sized mining facility.
- No tailings pond: Much of the sand contained in the oil sands deposit is left in the ground and never comes to surface. In-situ operations therefore do not require large tailings storage ponds and have no need for reclamation.
- Lower cost: Since there is no mine or tailings pond, in-situ operations require much less capital, are quicker to build, easier to bring on-line and generally less expensive to operate. These facilities can be built in phases and do not require the same economy of scale as surface mining operations.
- Lower bitumen recovery rates: Recovery rates for in-situ facilities can vary greatly, typically from 40 to 60%. Despite significant technological improvements in recent years, in-situ recovery rates are still much lower than mining operations which typically recover over 90% of the bitumen contained in the oil sands.
- Lower certainty: There is a larger degree of uncertainty in terms of positioning of the wellheads and expected recovery rates. Once steam is injected, it can take several months before a drop of bitumen is produced. This makes the economics of in-situ operations somewhat more variable, particularly in newly developed areas.
- More GHG emissions: In-situ extraction requires a large volume of steam to heat the bitumen in the ground. This steam is produced by burning natural gas. In-situ facilities therefore generate more greenhouse gas (GHG) emissions per barrel of bitumen produced as compared to an oil sands mining facility.
- Not easy to stop and start: In the event of an unexpected disruption, it is difficult to just shut-off steam, particularly in new facilities. Once steam is cut off to the well head, the reservoir will begin to cool. A very mature well (one that has been steaming for several years) will retain its heat relatively well and be much slower to cool. However, a new facility might cool down very quickly and take a long time to restart once steam is re-established. In contrast, mining facilities can be safely shutdown in less than a day and restored back to nameplate capacity within only few days.
IN-SITU VS MINING - ITS NOT A CHOICE: It is very important to stress that the reservoir actually dictates the bitumen extraction technique used. Reservoirs that are too close to the surface cannot be recovered in-situ since the risk of steam blow-out would be too high. Deposits that are too deep cannot be mined since too much overburden would have to be removed, making the process uneconomical. The ideal in-situ reservoir sits about 200 meters below the surface, while mining operations are typically within 50 meters from grade. Reservoirs that are in the middle (too deep to be mined but too shallow to be recovered in-situ) lie in a "no man's land" where neither process will work.
COMMERCIAL OPERATIONS
Advances in technology have greatly improved reliability and bitumen recovery rates for in-situ operations. In fact, in-situ bitumen production is expected to surpass production from surface mining in the next few years. There are 2 common thermal in-situ techniques employed in the oil sands:1. Cyclic Steam Stimulation (CSS)
2. Steam-Assisted Gravity Drainage (SAGD)
Both CSS and SAGD have identical central processing facilities. The difference lies in the positioning and number of wellheads.
1. CYCLIC STEAM STIMULATION (CSS)
- A single well is drilled into the oil sands deposit.
- High pressure steam is injected into the reservoir to heat the bitumen and reduce its viscosity. This steam continues to be injected for several weeks in order to fully saturate the reservoir.
- The bitumen is then allowed to soak for several days or weeks in the hot pressurized reservoir.
- As the reservoir cools, this provides the driving force to bring the oil up to the surface. The flow is then reversed so that the bitumen/water emulsion can be pumped back to the surface. This production phase can last for several weeks.
- At the processing plant, the water is removed from the bitumen, treated and recycled back into the process. The bitumen is sent to an upgrader for further processing or diluted and sold directly to market.


Imperial Oil's Cold Lake facility was the first in Canada to commercialize CSS recovery of bitumen. Initial recovery rates were only 20-25%, but recent improvements in technology have boosted recovery rates into the 50% range for some operations.

RECOVERY RATES AT COLD LAKE (COURTESY IMPERIAL OIL)
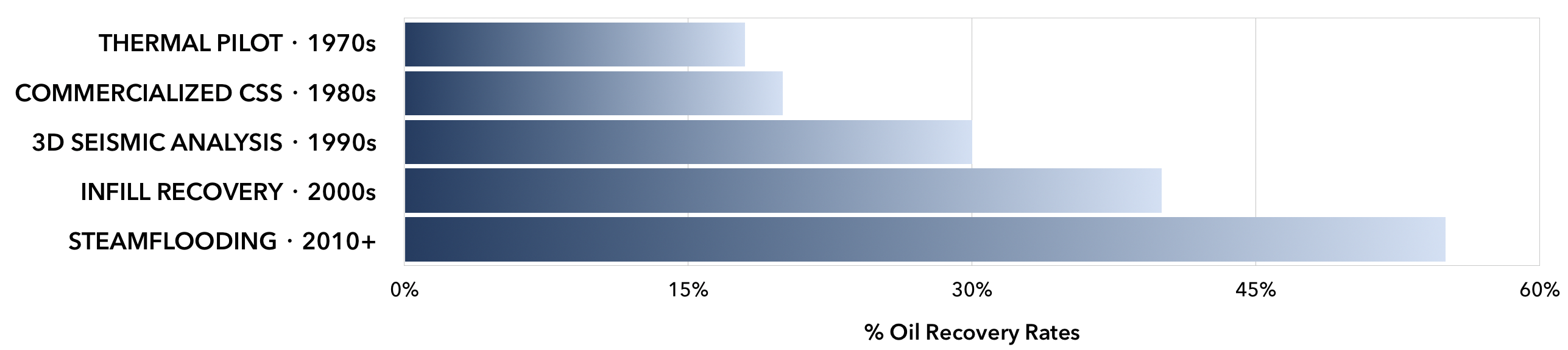
2. STEAM-ASSISTED GRAVITY DRAINAGE (SAGD)
- Two horizontal wells are drilled approximately 5 meters apart, one above the other. Well depth can vary anywhere from 150 to 450 meters. Each well can be up to 1,000 meters long.
- High pressure steam is injected into the top well, or the injectionwell. The hot steam heats the surrounding bitumen.
- As the bitumen warms up, it liquifies and begins to gravity flow to the lower well, or the producingwell.
- The bitumen and condensed steam emulsion contained in the lower well is pumped to the surface and sent to a processing plant, where the bitumen and water are separated.
- The recovered water is treated and recycled back into the process. The bitumen is sent to an upgrader for further processing or diluted with condensate and sold to market.


CSS VS SAGD: Note that the choice between CSS and SAGD technology is dictated by the geology of the reservoir. The Cold Lake deposit lends itself better to CSS while oil sands in the Athabasca region responds better to SAGD.
WATER DISTRIBUTION CIRCUIT
Thermal in-situ oil sands facilities use water and natural gas for the generation of steam. The quantity of water required is much lower than an equivalent mining facilities - only 3 barrels of water is needed to produce 1 barrel of oil. However, water quality is very critical when used for steam generation. Water treatment is therefore a very important component of any in-situ operation. A typical in-situ facility recycles about 90% of the water used on site. Most of the fresh water supply (make-up water) is sourced from groundwater aquifers.
BITUMEN EXTRACTION IN-SITU: WHERE THE GROWTH IS
Throughout the 1990s and into 2000, much of the growth in the oil sands was focused on mining. However the past 10 years have seen major expansion of in-situ bitumen extraction, particularly in the Athabasca Region near the town of Fort McMurray.
IN-SITU PRODUCTION BY RESERVOIR • CLICK FOR LIVE CHART →
IN-SITU OPERATIONS
There are several dozen commercial in-situ operations and a handful of small demo plants scattered throughout central and northern Alberta. Facilities in the Cold Lake area commonly use CSS to extract bitumen while most other facilities use SAGD. With the exception of production from Firebag and MacKay River, most facilities produce a heavy diluted bitumen (or Dilbit) sold directly to market, without an intermediate upgrading step.Here's a list of the largest in-situ facilities currently in operation in the oil sands:
[GOOGLE MAP - IMAGE @ BOTTOM plus TABLE]
BITUMEN UPGRADING EXPLAINED
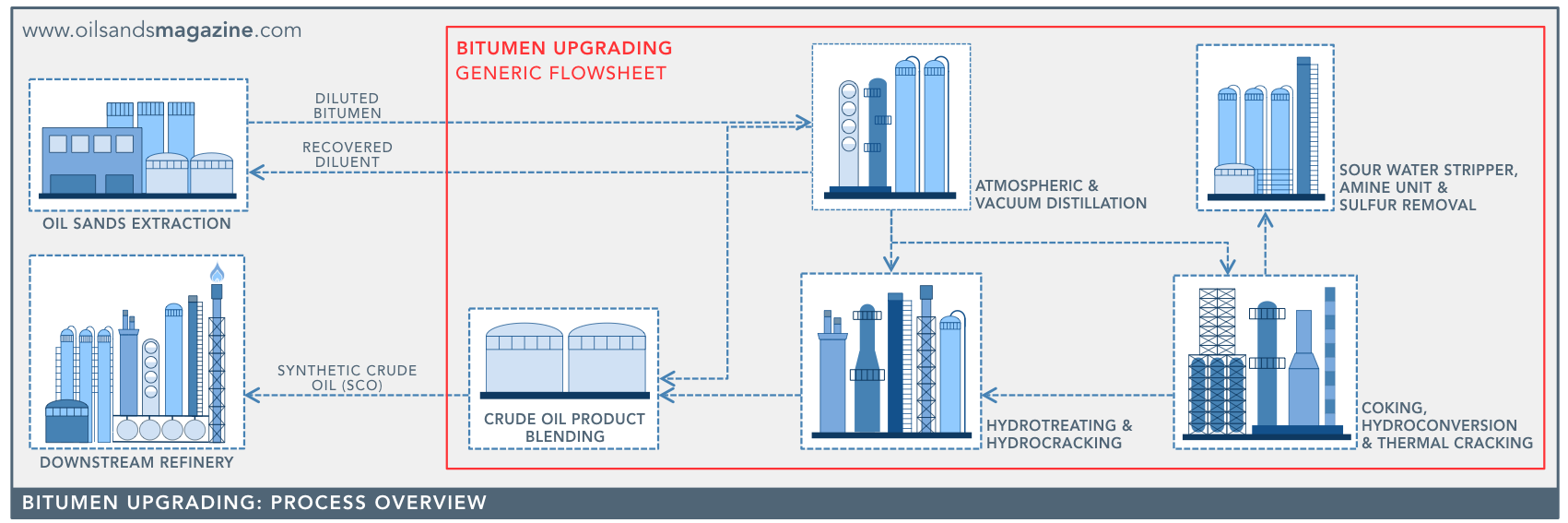
Part 2: Building Crude Oil Pipelines: How to Make Preliminary Cost Estimates
Published by: Rick Smead
The Shale Revolution sparked a multibillion-dollar re-plumbing of the U.S. crude oil pipeline network that continues to this day, two years after oil prices started falling and one year after oil production volumes followed suit. While the pace of development has at times seemed hectic, the individual decisions to build new pipelines involve a lot of studying, vetting and number crunching. After all, pipelines don’t come cheap, and their success depends to a considerable degree on their long-term usefulness to the market. One of the most important factors in determining whether a crude oil pipeline project makes sense is its capital cost and, with that, the cost of moving oil through it. Today, we continue our look at crude pipeline economics with a discussion of the basics of estimating pipe size and cost, and figuring the optimal capacity of a given pipeline project.
In Part 1 of this series, we discussed the long list of questions that midstream companies, potential shippers and others need to consider when deciding whether to build a new pipeline. Which production areas need new takeaway capacity? What should be the pipeline’s destination? How much capacity should the pipeline have? When is the ideal time to build the pipeline and bring it online? And –– also important –– how much would it cost to develop a crude oil pipeline from Point A to Point B, how much would the developers have to charge shippers, and would those charges be low enough for shippers to say, “We’re in.” We noted it is critical that developers consider future supply/demand dynamics to determine whether the need for capacity is likely to hold up. We discussed the facts that oil pipeline regulation is particularly complicated, and that transportation rates aren’t necessarily based on project costs. (More on that to come.) And we noted that this series on oil pipeline economics is a spin-off of sorts from our But I Would Pipe 500 Miles series on the economics of natural gas pipelines. As in that effort, the aim here is to present simple, rule-of-thumb approaches to determining whether an oil pipeline project is worth pursuing, not the detailed engineering analysis that would be needed. Today we consider the basics of estimating how big a pipe needs to be for a given level of oil transportation and what that size pipe will likely cost (including “non-pipe” elements like pumps, meters, and storage
So the first question is this: Say you want to move 200,000 barrels a day (200 Mb/d) of crude out of the Bakken play in North Dakota, and deliver it 500 miles. How fat –– or what diameter –– does the pipe need to be? We’ll go through the basic geometry and math, then give an industry rule of thumb and test it against our geometry lesson. There are three steps to determining the pipe diameter needed:
Step 1: Unit conversion. Pipe diameters are measured in inches, pipeline capacity is in barrels a day, and the speed of the oil through the pipe is in miles per hour. So somehow, we have to turn everything into the same units to back down from barrels per day to inches of diameter.
Step 2: We just mentioned the speed of the oil through the pipe—so we need to find and confirm what assumption is reasonable.
Step 3: We need to go through the math to get a basic formula.
Here we go. Step 1. What about the unit conversion? How many cubic feet per hour are there in a barrel per day? Well, a barrel of oil is 42 gallons. A gallon is 0.134 cubic feet. That means a barrel is 5.615 cubic feet, so a barrel per day is 5.615 cubic feet per day. There are 24 hours in a day, so 5.615 cubic feet per day is 0.234 cubic feet per hour. A pipe to move 200 Mb/d is trying to move 200,000 of those, so it’s 200,000 times 0.234, or 46,800 cubic feet per hour. What we want to know next, as shown in Figure 1, is how fast does the oil move, and how wide does the pipe need to be?
Step 2: How fast does the oil move? This can depend on a lot of factors. How thick is the oil? As we’d expect, light Bakken crude should move about like condensate –– pretty easily, that is ––but heavy stuff, like Canadian bitumen thinned out just enough to move by mixing in diluent, will be slower. How many pumping stations do we have, and where are they located along the route? Are any special lubricants being used, to “oil up the oil,” helping it move faster through the pipe? We could struggle with all of those questions, or we could look around for an industry rule of thumb, and then test it against currently pending projects. The industry rule of thumb regarding the speed of oil through a pipeline — some are faster, some are slower, but this is typical — is 4 miles per hour, or the pace of a brisk walk. For those of you who read our gas pipeline series, you’ll know that gas moves at about 20 miles per hour, so oil — being a liquid and a lot heavier than natural gas –– moves a lot more slowly. Now, since everything we’ve been converging on so far is in feet or cubic feet per hour, we need to convert 4 miles per hour to feet. At 5,280 feet per mile, this comes out to 21,120 feet per hour, moving down the pipe.
Now we come to Step 3. If we need to move 46,800 cubic feet per hour, and our crude oil is moving 21,120 feet in that hour, how wide does the pipe need to be? It needs a cross-sectional area big enough for 46,800 cubic feet to move 21,120 feet, or an area of 46,800 divided by 21,120, or 2.22 square feet. Going back to high school geometry, we know the area of a circle is the radius squared times Pi (or 3.14159…), so if we divide 2.22 by Pi, giving us 0.705 square feet, that gives us a radius of 0.84 feet, which is almost exactly 10 inches. So the diameter is two times that, or 20 inches.
Whew. Is that enough math for you? Which brings us to the industry rule of thumb. If all we did in the first place was to take the thousands of barrels per day (200), multiply it by 2 to get 400, then take the square root of that — 20, we have the same diameter it just took three steps and about 500 words to get to. So the bottom line is that the rule of thumb formula, as long as you can assume the typical speed through the pipe of 4 miles per hour, is this:
As noted, this all depends on the assumption as to the velocity of the oil through the pipe. So one last check is to ask just how “typical” the 4 miles-per-hour assumption is. Using our Midstream Infrastructure Database Interface (MIDI), we looked at 24 operational, under-construction, or still-active new-build crude oil projects across North America. The velocity implied by their diameters and capacities varied over a range about 4 miles per hour wide, but the average of the whole group was 4.02 miles per hour. Thus, there’s no question that the rule-of-thumb velocity is a good starting point for rough size and cost estimation.
So now we want a 20-inch pipe to move light crude 500 miles. This brings us to the next question, namely, what’s it going to cost to build? Even if we have no interest in building the pipeline ourselves, we need to know how much money a developer would have to raise and commit to do it to provide us with service. And ultimately, as we’ll get to in more detail in the next installment of this series, most ways of pricing transportation rates start with cost somewhere in the equation—even for unregulated pipelines. The most common rule of thumb for a rough estimation of pipeline capital cost is the cost per “inch-mile.” It’s a pretty simple concept: The longer and fatter the pipe is, the more it’s going to cost, so the length in miles is multiplied by the diameter in inches, to produce “inch-miles” to multiply by that unit cost.
Of course, there are a lot of reasons this cost per inch-mile can vary. For one example, rocky ground is more expensive to work with than nice soft prairie soil. For another, Beverly Hills is a lot more costly to pipe under than is open ground in Texas. (Don’t laugh — San Joaquin crude oil in California has to get down to Los Angeles refineries somehow.) And, of course, the configuration of pipe, pumps, offtake points, meters, etc. on different pipeline projects can vary by a lot. One last thing: The total cost of a project isn’t necessarily directly proportional to diameter, since a right-of-way is a right-of-way and the same piece of equipment that can pick up a 16-inch pipe can probably pick up a 20-inch pipe. But within similar configurations and roughly similar sizes of pipeline, the cost per inch-mile is usually pretty consistent. And the additional cost for pumps, meters and contingencies is also usually pretty constant as a percentage of the pipe cost. The one exception to all of this is tankage, since it is primarily a function of the amount of oil flowing, not how far it has to flow.
In a lot of small-diameter, short-pipe situations all over the producing areas, the cost per inch-mile should run about $60,000, before adding 15% for valves and meters, and another 10% for contingencies. Tanks run about $30 to $50 per barrel of storage, and are generally designed for three days of storage at full pipeline capacity. So in a simple example, a 50-mile, 6-inch-diameter line would have 50 times 6, or 300, inch-miles. At $60,000 per inch-mile, that would amount to $18 million for the line, or $22.8 million after adding 15% for valves and 10% for contingencies. A 6-inch pipe would have a capacity of 18,000 bbl/d (remember, 6 squared, divided by 2, times 1,000 –– the flip-side of the sizing formula above). The tankage requirement would be 54,000 bbl (or three days times 18,000 bbl). At $30 per barrel, that would be $1.6 million, or $2.0 million after gross-up. So the total cost of everything should be $24.8 million.
But what about our big, 20-inch, 500-mile pipe to bring Bakken crude to the world? A more typical cost per inch-mile for these big pipes is $75,000, and tankage is more likely to be $50 a barrel. So by going through the same calculation, 20 inches times 500 miles is 10,000 inch-miles, which would be $750 million at $75,000 per inch-mile. Multiply that by 1.15 for valves and meters, then multiply the whole thing by 1.1 for contingencies, and the pipeline cost is $949 million. Add 600,000 barrels of storage (remember, the capacity was 200,000 Bpd, and we need three days’ worth), at $50 per barrel, and we have a tank capital cost of $30 million. Gross it up by the same valve-and-meter and contingency factors, that gives us $38 million, which brings the total cost up to $986 million. Wow, it’s getting easier to spend a billion bucks all the time.
Can this all be turned into one big formula? Certainly. First, as interesting as the whole tank thing was, it’s only going to be about 4 or 5 percent of the cost of the line for one of the big guys, about 9 or 10 percent for a short line. So why not just build it into a formula, since this is a rough estimate in the first place. For a big, long line, let’s just add 4.5 percent to the line cost.
Ultimately then, here’s the formula:
Remember, the long way, we got $986 million. Is this close enough to avoid brain damage? We think so.
As we said, the cost per inch-mile and cost per barrel of tankage can vary a good bit, with the variation in the cost per inch-mile being the big one to worry about. So before relying on a formula like this, be sure to check how it works for some other pipelines in the same area or route, of about the same size. We’ve run it roughly against a sampling of MIDI projects, and our answers were in the middle of the CAPEX levels they report, so it’s a good rule of thumb. Next, we’ll be explaining the many strange and wonderful ways this estimate can turn into transportation tariffs.
Published by: Rick Smead
Many factors are weighed before a midstream company commits to building, or a shipper commits to shipping on, a major crude oil pipeline. Where is incremental pipeline capacity needed? What would be the logical origin and terminus for the pipeline? What should the project’s capacity be, and what would be the capital cost of building the project? Where the economic rubber really meets the road is the question of what unit cost––or rate per barrel––would the pipeline developer need to charge to recover its costs and earn a reasonable rate of return on its investment. Today we continue our review of crude oil pipeline economics with a look at the rules-of-thumb for determining what pipeline transportation rates would be.In Part 1 of this series we discussed the fact that new pipeline development is driven by either need or opportunity, and more often than not, a combination of the two. The key question that pipeline developers and their customers (the shippers) have to consider before committing to build new capacity, we said, is whether it will “pay” to flow crude on the pipeline once it’s built––not just the first year or the first three, but for years if not decades to come. To answer this question, pipeline developers and shippers have to consider both current and future economics. There are three fundamental factors that drive pipeline economics: 1) future supply dynamics (and the resulting price impact) at the origination point (Point A); 2) future demand (and price) at the destination point (Point B); and 3) the transportation cost to flow crude from Point A to Point B. In Part 2, we focused on estimating capital costs. We went through a geometry exercise to confirm an already-popular industry rule-of-thumb for estimating the diameter of the pipe to reach a certain capacity. We also explained and used a “cost per inch-mile” approximation to figure out what the pipe itself would probably cost, then included standard rough estimates of the cost for everything else: the pumps, storage, and meters necessary for the pipeline to work. We confirmed those numbers by reviewing some pending new projects, and ultimately got to the example of a 200-Mb/d crude oil pipeline 500 miles long costing $990 million to build.
Since we’ve now been in “rule-of-thumb” country for a while, that would be the best place to look for these answers as well. A typical developer would want to work out representative numbers to fill in all those operating-cost blanks: the staffing of the pipeline; the average salary and benefits for employees; the maintenance cost per mile; property and any other local taxes; the variable operating costs (such as pump electricity) that change with actual throughput; and the administrative overhead that would have to be covered. (As we indicated, there might be additional costs for a heavy-crude pipeline: to heat the stuff, and or to add diluent or special lubricants. These heavy-crude issues are so case-specific that we won’t clutter up our rule-of-thumb with them.) Even without the complication of enabling heavy crude to move through the pipe, it’s still a lot of factors to consider. To streamline the process, we’ve reviewed the results of the estimates of some of our favorite pipeline developers and made two significant conclusions: 1) fixed operating costs have a reliably consistent relationship with a pipeline’s capital cost (which, of course, is largely a function of the project’s length and capacity); and 2) the variable operating costs generally fall within a narrow cents-per-barrel range.
One more thing about operating costs––detail doesn’t matter much, because for any new pipeline, the impact of operating costs on the total annual revenue requirement is always a lot less than the impact of recovering the capital cost of the pipeline itself at a decent internal rate of return (IRR). In other words, don’t sweat the small stuff when the rule-making thumb is at work. Taking all of this into account and breaking out variable cost separately, the “first-look” quick estimate of the annual fixed operating costs that will need to be charged can reasonably be expected to be between 6 and 7% of the original capital cost—let’s call it 6.5%. And that variable cost we broke out separately is typically 30 cents per barrel, if the thick-crude issues we mentioned before aren’t taken into account.
Now, we take on those capital cost recovery and IRR issues. To do that, we need to determine the period of time over which we would like to recover our capital costs, as well as establish a reasonable IRR. For crude oil pipelines, the capital cost recovery period typically ranges from 10 to 20 years––it all depends on how strong the developer believes the market will be, or the type and length of contracts that are expected to be signed. In the case of the kind of large-capacity, long-distance pipeline we use as an example here, we’re going to assume that no one would go through the trouble of building the project if the business case for it were going to last less than 20 years. Meanwhile, as we’ll discuss in the next installment of this series, regulators will usually force capital cost recovery periods that are even longer––as long as 35 years. So let’s use 20 years for our rate wish-list. For a cost of money, the most typical IRR we’ve seen is 10%. Is this before taxes or after taxes? Yes. That is, there’s not another adjustment for income tax costs, but in a lot of cases pipeline developers are able to sort all of that out later, whether through the way their companies are structured—either as flow-through entities such as master limited partnerships (MLPs – see Masters of the Midstream), or as high-leverage (high-debt) investors with a lot of tax deductions. In other words, don’t worry about income taxes here, we’ll address them again in our regulatory explanation.
The end result is that we need an annual revenue level that will reimburse all the operating costs, pay off the original investment over 20 years, and earn a 10% IRR. That can just be a constant annual revenue level, designed like a house payment plus expenses. So we run a payment formula with a “rate” of 10%, a “period” of 20 years, and a “present value” of the original capital cost—in this case, $990 million. Then we add 6.5% of that capital cost to the answer for fixed operating expenses, to arrive at the dollars the pipeline needs to charge in a year to achieve its 10% IRR.
Using the Excel “payment” function, the capital-related annual payment looks like this:
= PMT (10%, 20, $990,000,000) = $116,285,029
Adding in fixed operating expenses at 6.5% of the capital investment of $990 million, the total revenue target for fixed costs looks like this:
Operating Expense = 6.5% X $990,000,000 = $64,350,000
+ $116,285,029 for Capital Recovery and Time Value
= $180,635,029 Total Revenue Target (round to $181 million)
The variable cost, already stated as 30 cents per barrel will be added at the end. So for the last step, turning all of this into a rate, it goes like this: At 200 Mb/d, full (100%) use of the pipeline would mean it would move 73 million barrels a year (200 Mb/d x 365 days). So if the shippers were expected to use the pipeline all the time at full capacity––or if the pipeline had agreements where they pay the rate as if they’re doing so––the rate would be:
$181 million divided by 73 million barrels, or $2.47, plus $0.30 variable cost = $2.77 per barrel.
But if the shippers will just pay as they go, based on actual use, we have to estimate how often they’ll really use the pipeline. A typical assumption is that they’ll use it at 60% percent of capacity, or a 60% load factor. So now, the annual volume is 73 million barrels times 60%, or 44 million barrels. So now the rate would look like this:
$181 million divided by 44 million barrels, or $4.11, plus $0.30 variable cost = $4.41 per barrel.
So depending on the market conditions and contract structures you assume, that would be the range$2.77 to $4.41 per barrel––to make the developer whole for the investment and out-of-pocket expenses over a 20-year life.
Published by: Rick Smead
Many factors are weighed before a midstream company commits to building, or a shipper commits to shipping on, a major crude oil pipeline. Where is incremental pipeline capacity needed? What would be the logical origin and terminus for the pipeline? What should the project’s capacity be, and what would be the capital cost of building the project? Where the economic rubber really meets the road is the question of what unit cost––or rate per barrel––would the pipeline developer need to charge to recover its costs and earn a reasonable rate of return on its investment. A really important aspect of that is what the developer will be allowed to charge, once regulators get into it. Today we continue our review of crude oil pipeline economics with an overview of who regulates oil pipelines, how they do it, and what it means for rates.In Part 2, we focused on estimating capital costs. First, we went through a geometry exercise to confirm an already-popular industry rule-of-thumb for estimating the diameter of the pipe to reach a certain capacity. Then we explained and used a “cost per inch-mile” approximation to figure out what the pipe itself would probably cost, inclusive of all the other stuff—pumps, storage, and meters—that go with the pipe. We confirmed the numbers based on some real-world projects, and ultimately estimated that our hypothetical 200 Mb/d pipeline, 500 miles long, would cost $990 million. The next step, in Part 3, was to figure out how much the developer would need to charge to pay off that $990 million pipeline and earn a decent internal rate of return, or IRR. The answer was a range of $2.77 to $4.41 per barrel, depending on how much oil moved and how the contracts were structured.
But now, we come to a crucial question: Will the developer be able to charge that much, if a Federal or state regulator gets to review the rates? What does it mean for the pipeline to be regulated? For the pipeline developer and owner, it can be a bunch of new hoops to jump through and keep jumping through, depending on who does the regulating. For the shipper, it can be a whole bunch of extra tools to get bargaining leverage with the pipeline, or even just to force the rates down over the life of a contract. But meanwhile, if regulation is too unfriendly, too likely to deny the pipeline owner the ability to make enough money, it can keep anyone from wanting to build the pipeline in the first place.
The impact and mechanics of rate regulation depend a lot on who does the regulating. We’ll focus most heavily on Federal regulation, since it’s the most complicated. Very simply, interstate pipelines, that actually cross one or more state lines, get regulated by the Feds. Which Feds? The Federal Energy Regulatory Commission, or FERC, the same agency that regulates natural gas pipelines. But that is where any similarity ends. FERC regulation of oil pipelines is based on the Interstate Commerce Act, the same law that governs trucks and other forms of interstate transportation, whereas natural gas pipelines are regulated according to the Natural Gas Act—a law crafted just for them. Up until 1977, oil pipelines were regulated by the Interstate Commerce Commission (ICC), which had its own pretty simple ways of doing things. The ICC let a pipeline set rates to recover its out-of-pocket costs, pay for depreciating the pipe, and earn a return on a “valuation” rate base.” We don’t need to get into that here, since it’s long-gone.
In 1977, through the Energy Organization Act, the Federal Power Commission was turned into the FERC, the Department of Energy was created, etc. Regulation of oil pipelines was moved from the ICC to the FERC, which also had its own way of doing things. The next couple of decades saw various changes by FERC and some incredibly complicated (and boring) ways of dealing with the transition. Since today the rules are pretty well settled, and especially for a brand-new pipeline none of that tortuous history matters much, we will keep it to ourselves. Besides, we never want to put you to sleep on your keyboard only to find a bunch of G’s in the middle of an email thanks to nose typing.
We will, however, arm you to be able to sound knowledgeable if you’re ever at an oil-pipeline cocktail party. Just remember:
Opinion 154B, in 1985: “Wasn’t 154B when FERC really took hold and pushed its own way of doing things on oil pipelines?”
Energy Policy Act of 1992: “Good thing Congress streamlined things in EPAct92 and let FERC give us some consistent rules. Wow, ‘Congress’ and ‘streamlined’ in the same sentence—I must be dreaming.” And
Order No. 561 in 1992: “Yeah, when they really made indexing the primary way to set rates, it sure saved a lot of uncertainty and lawyer costs.”
Then, if anyone presses you, say, “That’s all ancient history” and count on any further discussion to lose the audience anyway. If you want to know much more detail, go to an Association of Oil Pipelines meeting sometime and get the full story.
But now back to the present: When an interstate oil pipeline starts shipping oil for third parties—whether it’s a new pipeline or an existing pipeline that previously just shipped its owner’s oil, it has to file a tariff laying out rates that will apply equally to each type of customer. Those rates are capped at either a rate agreed upon by a shipper not affiliated with the pipeline, or by a cost-of-service study. There are other options for setting rates once the pipeline is in service—most notably “market-based” rates, if the pipeline can prove lack of market power, where there are a ton of other issues. But we won’t get into them here, we’re just trying to decide whether to build the thing in the first place.
What’s a cost-of-service study? It’s the same thing any regulated utility (or gas pipeline, for that matter) does in a rate case. The rates are based on the cost of doing business:
- Variable operating costs like pump electricity, that follow the level of throughput;
- Fixed out-of-pocket costs (costs that don’t follow throughput) like payroll, rent, maintenance, and taxes like property taxes that aren’t based on income;
- Payback of the facility cost (depreciation);
- Return on the net investment after depreciation, based on a mix of debt and equity funding: and
- Income taxes based on the profit built into the return.
Then the total cost of service gets divided by the expected billed throughput. This can be where we get into two different kinds of shippers: “Committed” and “Uncommitted.” Frequently shippers who have committed to long-term contracts get billed as if they took their full contract every day.
Uncommitted tend to be the rest of the industry, paying as they go. So, for example, if a pipeline were half committed and half uncommitted, and the uncommitted shippers were expected to fill 60 percent of the uncommitted capacity, the “billed throughput” would be the full committed contracts at 100 percent, and uncommitted at 60 percent, for an overall load-factor, or billing level, of 80 percent of the total capacity.
So let’s run through an example of FERC’s rate setting methodology, based on the capital cost, operating cost, and range of throughput we worked out for the pipeline developer’s economic target in Part 3 of this blog series:
Variable Operating Cost: 30 cents per barrel
Fixed Cost of Service
Fixed Operating Cost: $64,350,000 (this is from Part 3
Depreciation: $33,000,000 (Capital cost of $990 million over a typical FERC 30-year life)
Return on Investment: $74,250,000 (7.5% return, based on a 10% return on equity, less 1% inflation, and interest on debt of 6%, equity and debt weighted at 50% each of the capitalization—but don’t sweat it, it’s reasonable)
Income Taxes: $37,867,500 (tax on the return on equity piece, plus tax on the tax)
Adjust for FERC Rules: ($1,485,000) (FERC requires a reduction in the investment being earned on, to reflect half a year’s depreciation and deferred income taxes—don’t ask—which is practically worth only 15 hundredths of a percent of the capital cost)
Fixed Cost of Service: $207,982,500 (Note that all of these items are based on a percentage of the capital cost—even fixed operating cost, which was estimated at 6.5 percent of capital cost back in Part 3. So if you add up the percentages, it comes out to about 21 percent—so you can just cut this whole thing short by multiplying the capital cost by 21 percent—we just wanted to show you why, before we gave you the quick way.)
Using the 200,000 barrel per day capacity and the 80 percent average load factor we explained above, the total billed throughput in a year would be 200,000 X 80% X 365 days, or 58,400,000 barrels per year.
Dividing the fixed cost of service by that, and adding back the 30 cents per barrel variable cost, the rate would be $3.86 per barrel.
Remember, the range of rates we got in Part 3, to come up with what would probably make a developer happy? It was $2.77 at 100 percent load factor, to $4.41, at a 60 percent load factor. The average of those two, which would be the answer at the 80 percent load factor we’re assuming here, would be $3.59 per barrel. So the bottom line to all of this agony is that, at least initially, typical FERC ceiling rates would actually be higher than what the developer needed to achieve a 10 percent internal rate of return over ten years.
So far so good. But the developer was counting on a constant rate over ten years, not one that trails off over time, the way cost-of-service rates do. So what happens next?
What happens next is indexing. Under FERC’s rules, once the initial ceiling rates are set, the pipeline gets to or has to adjust them according to inflation based indices FERC publishes once a year (see Figure 2). This keeps the regulated rate cap not only flat, as the Part 3 formulation did, it lets it increase, although from time to time it makes it decrease a little.
Figure 2 - FERC Oil Pipeline Index
(Per FERC Regs at 18CFR342.3)
Over the last ten years, these annual index postings have allowed oil pipeline rates to go up by a combined 44 percent, meaning that the rate level the developer would need, starting 27 cents below the cap and getting more headroom every time the index goes up, should be very safe from being capped by a regulated rate.
But wait—naturally, there are still a couple of flies in the ointment for the developer. First, as with everything else we’ve done in this series, our regulated ceiling estimate is based on typical results, “rules of thumb,” and in any individual case every piece of the cost of service and throughput denominator might get beaten up by FERC staff and intervenors if they decide to make an issue of it. Second, there is always the looming risk that FERC will call in the developer to true up to a new cost of service later. Oil pipelines have to report their approximate cost of service every year in FERC Form 6, on “page 700” (page 700? Yes, regulation comes with a lot of paper). If the cost of service is a lot lower than the indexed rate, FERC can reduce the ceiling. And third, the rules can change. Remember that “income tax” allowance, about 18 percent of the fixed cost of service? Twice, as recently as this July, the US court of appeals has told FERC to go back to the drawing board in allowing it, since most oil pipelines are part of master limited partnerships (MLPs) that don’t pay income taxes themselves—they flow the liability through to their owners-partners. So the industry has to keep fighting to maintain that major chunk of cost of service in its rate ceilings, and the risk of losing it is always there.
For crude oil pipelines, it’s often not enough to get along with their shippers and keep FERC activity from going on. As we indicated back in Part 1, the same rules apply to refined-product pipelines, who have customers such as airlines, and thus usually end up in a lot more litigation. When those pipelines lose a big policy decision (like the tax allowance), it applies to everyone. So regulation does always include some risk. For the shipper, though, regulation means that there is always someplace to go if the shipper is unhappy with the rates.
So that’s our whirlwind tour of Federal rate regulation. Pipelines that never get outside of one state are regulated by state agencies (like the Texas Railroad Commission-TRRC, or the California Public Utilities Commission-CPUC). We mention those two because they’re the extremes of how states regulate crude oil pipelines. In Texas, unless shippers complain, the pipeline can usually make its deals with shippers and report the numbers to TRRC. (We are in the middle of a blog series on Texas intrastate pipelines that goes into a lot more detail on this. See Floodin' Down in Texas.) But in California, crude oil pipelines are regulated as utilities, just like the local gas or electric company, with very strict, rigid, and low cost-of-service standards. So it’s much harder to make a decent return on a crude-oil pipeline in California than it is in Texas. Other states fall in broad ranges in-between the two.
Wrapping up our series, we hope we’ve given you enough to estimate when and how a crude-oil pipeline can make economic sense, and whether to worry much about regulation. The other pieces of the puzzle—will there be enough oil long-term to support the pipeline, will it be more economic over time than rail, and will the price basis differential hold up for the life of the pipeline—take a lot of review of all the work RBN does around crude oil markets in the United States. So for now, our rule-making thumb will rest.
New Crude and Diluent Pipelines and Storage Capacity in Alberta
Several oil-sands expansion projects committed to when crude oil prices were double what they are today are finally coming online, and midstream companies active in Alberta are building new crude/diluent pipelines and storage capacity to keep pace. New storage caverns for natural gas liquids are also in the works, giving a much-needed boost to Canada’s Energy Province. Today we conclude our series on midstream infrastructure under development in or near Western Canada’s oil sands region that move and store hydrocarbon liquids.
Alberta––sometimes called “the Texas of the North”––has experienced as many ups and downs in its oil patch as the Lone Star State, and lately, with crude prices hovering near $50/bbl and the Fort McMurray, AB area still rebuilding from the wildfires of May 2016 (see Back in the High Life and Over the Hills and Far Away), it’s fair to say that times have been better. The good news is that oil prices have been inching up; a number of the projects committed to a few years ago to boost oil sands production are approaching commercial operation; and the expected output increases are spurring the development of new midstream infrastructure. In Part 1 of this series, we discussed the challenges that oil sands producers have faced (low crude prices, the wildfires, the extra costs associated with moving bitumen and heavy oil to market, etc.), as well as the fact that Alberta producers decided a few years ago (when Western Canadian Select – WCS - crude prices topped $70 to $90/bbl) to undertake a combined 850 Mb/d or so of oil sands capacity-expansion projects that would come online in the 2015-19 period. Those projects have had a domino effect, resulting in the need for a lot more diluent––either in the form of natural gasoline (a natural gas liquid, or NGL) or field condensate (superlight crude oil)––to decrease the viscosity of bitumen and heavy oil and enable them to flow through pipelines. In Part 2, we discussed how the need for more diluent has encouraged natural gas producers in Alberta to focus on “wet” gas and field condensate production in the province’s Montney and Duvernay plays, and how that shift has spurred the development of new natural gas processing capacity (to extract NGLs and remove impurities from raw gas) and fractionation capacity (to separate mixed NGLs into purity products like ethane, propane, butane and natural gasoline––the leading diluent material). Taken together and if they all happen, the Alberta projects we discussed would add 163 Mb/d of fractionation capacity and 800 MMcf/d of natural gas processing capacity; projects just over the border in British Columbia would add another 1 Bcf/d of gas processing capacity.
This time it’s all about new pipelines and storage being built within Alberta’s borders. The aims of these projects (like a lot of midstream work) are a combination of catch-up and in-anticipation-of––that is, the new infrastructure will address current shortfalls in pipeline and storage capacity and provide the incremental midstream capacity that the new oil sands (and other) production will require when the expansion projects we discussed in parts 1 and 2 come online. We begin with Alberta pipeline projects, the longest of which is the 286-mile Grand Rapids Pipeline (blue dashed line in Figure 1), which will actually consist of two parallel pipelines flowing in opposite directions between Edmonton, AB and an oil sands production area northwest of Fort McMurray.
Figure 1: Planned Alberta crude and diluent pipelines; Sources: RBN and company presentations
A 20-inch-diameter pipeline will transport up to 225 Mb/d of diluent north from Edmonton to the oil sands area near Fort McMurray, and a 36-inch-diameter pipeline will move up to 900 Mb/d of diluted bitumen or “dilbit”––a blend of diluent and bitumen (see Heat It for more on dilbit) from the Fort McMurray/oil sands area to Fort Saskatchewan (a half-hour’s drive northeast of Edmonton) for now and to Edmonton when market conditions warrant. All but the Fort Saskatchewan-to-Edmonton crude pipeline section will become operational in 2017. A 50-50 joint venture (JV) of TransCanada Corp. and Brion Energy owns most of the Grand Rapids Pipeline project; in August 15 the JV agreed to sell a half-stake in the 28-mile Edmonton-to-Fort Saskatchewan section of Grand Rapids’ northbound diluent pipeline to Keyera Corp., whose Edmonton terminal is the pipeline’s starting point.
The next-longest pipeline under construction in Alberta is the 269-mile Norlite Pipeline (purple line in Figure 1), a 70/30 JV of Enbridge and Keyera that will transport up to 218 Mb/d of diluent from Enbridge’s Stonefell terminal near Fort Saskatchewan to Enbridge’s Fort McMurray South terminal (which is located just where its name suggests), with a transfer line to Suncor’s East Tank Farm. The 24-inch-diameter Norlite project (also known as Line 74), whose capacity is expandable to 465 Mb/d, will come online in the first half of next year (2017). Enbridge, which in September 2016 unveiled a plan to merge with Spectra Energy, is planning a pair of 36-inch-diameter pipeline projects that together will move dilbit south from its Cheecham, AB terminal to Hardisty, AB. The first is the Wood Buffalo Extension (green line), which would––wait for it––extend Enbridge’s existing Wood Buffalo Pipeline (which runs from the company’s Athabasca Terminal near Fort McMurray to Cheecham, and which is also known as Line 75). The 65-mile extension (south to Kirby Lake, AB) will carry up to 800 Mb/d of dilbit when it starts up next year. The second 36-inch-diameter project is a “twinning” of the 214-mile portion of Enbridge’s Athabasca Pipeline (aka Line 19) from Kirby Lake to Hardisty. The Athabasca Twin Pipeline (orange line) will add 800 Mb/d of southbound dilbit capacity and is planned to begin operating by mid-2017. By the way, the combo of the Wood Buffalo Extension and the Athabasca Twin Pipeline will be known as Line 45.)
That’s a lot of new crude and diluent pipeline capacity, and it’s matched by a slew of planned Alberta storage capacity, not just for crude and diluent but for mixed NGLs, ethane and other purity NGLs.
They include:
- The Base Line Terminal, a new crude storage terminal that a JV of Kinder Morgan and Keyera is constructing at Keyera’s Alberta EnviroFuels site in Strathcona County, AB (near Edmonton). The facility, which will be connected to Kinder Morgan’s Edmonton-area storage and rail terminals, initially will have 12 crude storage tanks (white tanks to lower right in rendering below) with a combined capacity of 4.8 MMbbl but could be expanded by another 1.8 MMbbl (brown tanks to lower left). The first of the 400-Mbbl tanks are planned to be completed during the first half of 2017.
- Keyera’s plan to add 2.5 MMbbl of underground propane (and other NGL) storage capacity at its Keyera Fort Saskatchewan (KFS) facility by 2019 through the development of four new salt caverns at the site. (It already has 13 caverns there with a combined capacity of 12.5 MMbbl.)
- Expansions at Gibson Energy’s Edmonton and Hardisty crude oil/diluent terminals. At Edmonton, where Gibson already has 600 Mbbl of storage capacity, the company is adding a 300-Mbbl tank (for Statoil) and a 22-car rail loading facility that is slated to come online by the end of this year. Gibson also is planning to add two 400-Mbbl tanks by the second quarter of 2018 that are underpinned by a long-term, take-or-pay contract. The company has even bigger plans at its Hardisty terminal, where Gibson is adding 1.1 MMbbl of tank storage capacity by the end of 2016 and another 1.8 MMbbl by mid-2017.
- A joint effort by ATCO Energy Solutions and Petrogas Energy to develop a total of 2.5 MMbbl of underground storage for propane, butane and ethylene at four new salt caverns at ATCO’s Heartland Energy Centre near Fort Saskatchewan. Half of the capacity is scheduled to come online by the end of 2016, with the other half to follow in the first half of 2017.
As we said, these crude/diluent pipelines and crude/diluent/NGL storage facilities are being developed both to meet existing needs and to prepare the market for the new oil sands production capacity that will be coming online. Whether this round of Alberta’s midstream projects will be the last for a while will depend to some degree on whether we’ve seen the last of the big oil sands expansions. Producers have been working to improve efficiencies and reduce costs, but further investment in bitumen production can be a tough sell with crude prices at current levels.
IN THE NEWS
[Deborah Yedlin is a Calgary Herald columnist July 29 20176] Notes of caution dominated the conversations surrounding the release of second-quarter results by oilsands players on Wednesday and Thursday.
What was clear from the messaging coming from each of the three companies on the earnings calendar — Suncor, Cenovus and MEG — is that costs are coming down and the era of oilsands megaprojects is definitely over.
“The biggest takeaway is the success companies have had in bringing down their costs,” said Mike Dunn, director, institutional research with FirstEnergy Capital Corp. pointing to Cenovus and MEG.
The drop in costs — from changes in operating practises — is allowing companies to put the dollars saved towards modest growth opportunities, with Dunn expecting as much as two-thirds of what has been reduced should remain in place when prices do start to recover.
Supporting the perspective the time of megaprojects is over is the fact it is more attractive from a valuation perspective to buy oilsands assets because they are cheap. Other reasons for not turning on the spending taps include low prices, uncertainty over the impact of federal climate change legislation and the lack of progress on achieving access to tidewater; progress on pipelines remains a critical factor to companies making investment decisions — even in a low price environment.
And that’s despite the fact the capital markets, for the most part, have been open for the “good” names in the oilpatch and allowed for more than $15 billion to be raised in both the debt and equity markets since the beginning of the year. Not only that, there are signs overseas and transborder investors — even with the concerns that may exist over environmental issues — are starting to buy up shares in oilsands companies. The reason behind the renewed interest in the sector seems to be stemming from a sentiment that shares have bottomed, the supply response looms and investors with longer time horizons are looking to position themselves for big gains over the next 12-18 months.
Look no further than a company like MEG, which, for better and worse, is highly levered to oil prices and trading below $6 per share. A sustained jump in crude prices could see their shares respond in short order — especially if prices can stick in the US$60 per barrel range. If they do reach that threshold, MEG will be in a position to fund its expansion plans from internal cash flow; if prices remain closer to the $50 mark, analysts are speculating MEG will be going to the market to raise equity, but this could be deferred if it sells its interest in the Access Pipeline. Devon sold its stake to Wolf Midstream earlier this month for $1.4 billion.
The good news from MEG, though it wasn’t the “knock your socks off” variety, was that it is expecting to spend $30 million of the $170-million capital budget on growth opportunities; previously, the entire amount was to have been allocated to sustaining capital. Also interesting was that Bill McCaffrey, the founder and chief executive officer, is no longer chairman of the company. He has been replaced in the chairman’s role by Jeff McCaig. While this brings MEG into the modern age of corporate governance, it also sends a signal that the distance created by this move points to a greater possibility of a sale being considered if an offer comes forward.
Cenovus also had news of modest reinvestment plans, with the company in a position to capture the impact of achieving lower operating costs, and putting Phase G of Christina Lake back on the table after being shelved last year. The company is currently rebidding the project and between that and the operating efficiencies it says it has captured, Cenovus chief executive Brian Ferguson was clearly preparing the market that the company is looking to add to production.
But slowly.
Given the continued uncertainty in the markets — whether in terms of prices, access to tidewater or climate change policy — the comments made by Suncor CEO Steve Williams should not have been a big surprise.
Williams said the company is in discussions with the Alberta government to shut-in production in certain areas, which would result in the stranding of some assets in its portfolio.
Let’s chew on that for a bit.
The company said they would do this if the economics don’t work because prices are too low, or if the production at these sites would add to Suncor’s carbon emissions footprint.
It wouldn’t be the first time a company has shut-in production — though the fact it is in discussions with the province suggests it is a permanent move, because companies are granted permission to produce the entirety of reserves on Crown lands.
In other words, it’s a tad unusual on the part of the company. What usually happens is the shut-in reserves are brought back on-stream when commodity prices strengthen.
The fact it goes against current government policy also makes it a departure from the norm. One has to wonder whether the carbon footprint factoring into the equation is a thinly disguised ploy to get the government to agree to Suncor’s request, because of the government’s focus on reducing carbon emissions.
That said, it would be incorrect to think Suncor would be shutting in its marquee producing assets. Rather, the move would pertain to older wells, whose productivity has fallen but still require the same amount of steam to extract the bitumen; that’s not exactly efficient, cost-effective, or carbon friendly. Nor does it result in maximum utilization of its oil treatment facility.
There are valid economic reasons for a company to go down this route, especially in this capital-constrained world. However, the fact the carbon emissions argument could function as the deciding factor that convinces the government to go against existing policy could see more companies follow suit. However, industry and government choose to address this issue, it must be done thoughtfully and carefully because it would constitute a dramatic shift in policy direction; the last thing the industry needs is more uncertainty nor does the government want to be dealing with anything resembling unintended consequences.
What was clear from the messaging coming from each of the three companies on the earnings calendar — Suncor, Cenovus and MEG — is that costs are coming down and the era of oilsands megaprojects is definitely over.
“The biggest takeaway is the success companies have had in bringing down their costs,” said Mike Dunn, director, institutional research with FirstEnergy Capital Corp. pointing to Cenovus and MEG.
The drop in costs — from changes in operating practises — is allowing companies to put the dollars saved towards modest growth opportunities, with Dunn expecting as much as two-thirds of what has been reduced should remain in place when prices do start to recover.
Supporting the perspective the time of megaprojects is over is the fact it is more attractive from a valuation perspective to buy oilsands assets because they are cheap. Other reasons for not turning on the spending taps include low prices, uncertainty over the impact of federal climate change legislation and the lack of progress on achieving access to tidewater; progress on pipelines remains a critical factor to companies making investment decisions — even in a low price environment.
And that’s despite the fact the capital markets, for the most part, have been open for the “good” names in the oilpatch and allowed for more than $15 billion to be raised in both the debt and equity markets since the beginning of the year. Not only that, there are signs overseas and transborder investors — even with the concerns that may exist over environmental issues — are starting to buy up shares in oilsands companies. The reason behind the renewed interest in the sector seems to be stemming from a sentiment that shares have bottomed, the supply response looms and investors with longer time horizons are looking to position themselves for big gains over the next 12-18 months.
Look no further than a company like MEG, which, for better and worse, is highly levered to oil prices and trading below $6 per share. A sustained jump in crude prices could see their shares respond in short order — especially if prices can stick in the US$60 per barrel range. If they do reach that threshold, MEG will be in a position to fund its expansion plans from internal cash flow; if prices remain closer to the $50 mark, analysts are speculating MEG will be going to the market to raise equity, but this could be deferred if it sells its interest in the Access Pipeline. Devon sold its stake to Wolf Midstream earlier this month for $1.4 billion.
The good news from MEG, though it wasn’t the “knock your socks off” variety, was that it is expecting to spend $30 million of the $170-million capital budget on growth opportunities; previously, the entire amount was to have been allocated to sustaining capital. Also interesting was that Bill McCaffrey, the founder and chief executive officer, is no longer chairman of the company. He has been replaced in the chairman’s role by Jeff McCaig. While this brings MEG into the modern age of corporate governance, it also sends a signal that the distance created by this move points to a greater possibility of a sale being considered if an offer comes forward.
Cenovus also had news of modest reinvestment plans, with the company in a position to capture the impact of achieving lower operating costs, and putting Phase G of Christina Lake back on the table after being shelved last year. The company is currently rebidding the project and between that and the operating efficiencies it says it has captured, Cenovus chief executive Brian Ferguson was clearly preparing the market that the company is looking to add to production.
But slowly.
Given the continued uncertainty in the markets — whether in terms of prices, access to tidewater or climate change policy — the comments made by Suncor CEO Steve Williams should not have been a big surprise.
Williams said the company is in discussions with the Alberta government to shut-in production in certain areas, which would result in the stranding of some assets in its portfolio.
Let’s chew on that for a bit.
The company said they would do this if the economics don’t work because prices are too low, or if the production at these sites would add to Suncor’s carbon emissions footprint.
It wouldn’t be the first time a company has shut-in production — though the fact it is in discussions with the province suggests it is a permanent move, because companies are granted permission to produce the entirety of reserves on Crown lands.
In other words, it’s a tad unusual on the part of the company. What usually happens is the shut-in reserves are brought back on-stream when commodity prices strengthen.
The fact it goes against current government policy also makes it a departure from the norm. One has to wonder whether the carbon footprint factoring into the equation is a thinly disguised ploy to get the government to agree to Suncor’s request, because of the government’s focus on reducing carbon emissions.
That said, it would be incorrect to think Suncor would be shutting in its marquee producing assets. Rather, the move would pertain to older wells, whose productivity has fallen but still require the same amount of steam to extract the bitumen; that’s not exactly efficient, cost-effective, or carbon friendly. Nor does it result in maximum utilization of its oil treatment facility.
There are valid economic reasons for a company to go down this route, especially in this capital-constrained world. However, the fact the carbon emissions argument could function as the deciding factor that convinces the government to go against existing policy could see more companies follow suit. However, industry and government choose to address this issue, it must be done thoughtfully and carefully because it would constitute a dramatic shift in policy direction; the last thing the industry needs is more uncertainty nor does the government want to be dealing with anything resembling unintended consequences.
Oil sands output seen growing, need for new pipelines ‘urgent’: industry groupAdd to ...
Shawn McCarthy
OTTAWA — The Globe and Mail
OTTAWA — The Globe and Mail
Published Thursday, Jun. 23, 2016 8:46AM EDT
Last updated Thursday, Jun. 23, 2016 8:47AM EDT
Last updated Thursday, Jun. 23, 2016 8:47AM EDT
Canada’s oil sands sector is expected to add 1.55 million barrels per day between now and 2030 despite the depressed prices, and will require greater pipeline capacity to reach markets, the Canadian Association of Petroleum Producers said Thursday.
Basing its forecast on a survey of producers, CAPP expects the oil sands sector to add 850,000 barrels per day (b/d) between 2015 and 2021, largely as a result of projects begun before the 2014 price collapse.
Between 2021 and 2030, the oil sands producers would boost supply by 700,000 b/d, despite the cancellation or delay of numerous major projects over the past two years. CAPP reduced its 2030 forecast by 300,000 b/d from its 2015 outlook.
“The need to build new infrastructure within Canada is clearly urgent,” CAPP president Tim McMillan said in a release. “New pipelines will deliver more Canadian energy to Canadians, build our country’s economic prosperity and help Canada meet the world’s growing energy needs.”
The industry has several proposed pipeline projects at various stages of review. But the pipeline proposals have generated considerable controversy across the country, with local politicians, First Nations and environmentalists voicing staunch opposition to each of the projects aimed at bring landlocked western crude to Canadian coasts for export.
They include Enbridge Inc.’s 500,000 b/d Northern Gateway, which was conditionally approved by the Conservative government but remains stalled; Kinder Morgan Inc.’s proposed expansion of its TransMountain pipeline to Vancouver which the National Energy Board recommended be approved and is now undergoing further review by the Liberal government, and Trans Canada Corp.’s 1.1-million b/d Energy East line, for which the NEB will commence hearings in August.
The country’s pipeline network can move about 4-million b/d, which was roughly equal to the average production last year, the industry association said. There is also 754,000 b/d in rail loading capacity, a more expensive means of transporting crude than pipelines.
All told, the industry expects to boost crude production by 28 per cent over the next 15 years to 4.9-million b/d. That’s 400,000 b/d lower than the 2030 forecast issued last year. The oil sands will account for 3.7-million b/d of that 2030 total, CAPP said.
Basing its forecast on a survey of producers, CAPP expects the oil sands sector to add 850,000 barrels per day (b/d) between 2015 and 2021, largely as a result of projects begun before the 2014 price collapse.
Between 2021 and 2030, the oil sands producers would boost supply by 700,000 b/d, despite the cancellation or delay of numerous major projects over the past two years. CAPP reduced its 2030 forecast by 300,000 b/d from its 2015 outlook.
“The need to build new infrastructure within Canada is clearly urgent,” CAPP president Tim McMillan said in a release. “New pipelines will deliver more Canadian energy to Canadians, build our country’s economic prosperity and help Canada meet the world’s growing energy needs.”
The industry has several proposed pipeline projects at various stages of review. But the pipeline proposals have generated considerable controversy across the country, with local politicians, First Nations and environmentalists voicing staunch opposition to each of the projects aimed at bring landlocked western crude to Canadian coasts for export.
They include Enbridge Inc.’s 500,000 b/d Northern Gateway, which was conditionally approved by the Conservative government but remains stalled; Kinder Morgan Inc.’s proposed expansion of its TransMountain pipeline to Vancouver which the National Energy Board recommended be approved and is now undergoing further review by the Liberal government, and Trans Canada Corp.’s 1.1-million b/d Energy East line, for which the NEB will commence hearings in August.
The country’s pipeline network can move about 4-million b/d, which was roughly equal to the average production last year, the industry association said. There is also 754,000 b/d in rail loading capacity, a more expensive means of transporting crude than pipelines.
All told, the industry expects to boost crude production by 28 per cent over the next 15 years to 4.9-million b/d. That’s 400,000 b/d lower than the 2030 forecast issued last year. The oil sands will account for 3.7-million b/d of that 2030 total, CAPP said.
Take a master's level course in improving your energy literacy, down to the half a percent of our energy that we use to fly military jets. Saul Griffith likes numbers. The serial entrepreneur and MacArthur genius once calculated the carbon footprint of every single action in his life—from buying underwear to paying taxes. Now he and a group of colleagues at Otherlab, his San Francisco-based company, have mapped out something else in obsessive detail: all of the energy used in America.
"I think we may be the first three or four people to read every footnote in every energy agency document ever produced," Griffith said at a recent talk when he presented the new flowchart—which is still in a somewhat rough iteration—at an event run by Reinvent, a company that brings innovators together to talk about how to reshape the world.
The data is pulled from sources like the Department of Energy and the census. "This is really the first time that all of this data is brought together in one flow diagram, which is important if you really want to understand the consequences through the whole economy of things like defense," he says.
The left side shows where we get energy—solar, though growing, is still less than 1% of energy production. Most electricity comes from coal. For each source, it's possible to see how much is lost as waste at the other end; nearly half of the energy from natural gas, for example, ends up wasted.
By clicking through the chart, you can see exactly how much energy is used for every activity. Moving newspapers around uses a tenth of a percent of our total energy pie; driving trash to landfills takes about twice as much. Half a percent of U.S. energy is used to fly military jets. Almost 1% is used just to pump natural gas around pipelines; 2% goes to making cardboard and other paper products. Around 16% is used to grow, distribute, and cook food.
Looking at the total picture helps make the point that some of the ways that we think about energy aren't quite right. Refining petroleum uses about 6% of total energy in the country, but isn't considered when we think about fuel economy in cars. "If you think about it, every car you think is getting 40 miles a gallon is actually getting 30 miles a gallon, and maybe worse," Griffith said. Mining oil and gas uses even more energy.
The flowchart makes it clear that a huge amount of energy goes into infrastructure. "I think if you did a full accounting of this—what individual purchasing decisions do you make, what can you influence—I think you only influence about 40% of this," Griffith said. "To really solve this, we have to solve a lot of things at a deep infrastructure level. You need to be civically engaged."
"I think we may be the first three or four people to read every footnote in every energy agency document ever produced," Griffith said at a recent talk when he presented the new flowchart—which is still in a somewhat rough iteration—at an event run by Reinvent, a company that brings innovators together to talk about how to reshape the world.
The data is pulled from sources like the Department of Energy and the census. "This is really the first time that all of this data is brought together in one flow diagram, which is important if you really want to understand the consequences through the whole economy of things like defense," he says.
The left side shows where we get energy—solar, though growing, is still less than 1% of energy production. Most electricity comes from coal. For each source, it's possible to see how much is lost as waste at the other end; nearly half of the energy from natural gas, for example, ends up wasted.
By clicking through the chart, you can see exactly how much energy is used for every activity. Moving newspapers around uses a tenth of a percent of our total energy pie; driving trash to landfills takes about twice as much. Half a percent of U.S. energy is used to fly military jets. Almost 1% is used just to pump natural gas around pipelines; 2% goes to making cardboard and other paper products. Around 16% is used to grow, distribute, and cook food.
Looking at the total picture helps make the point that some of the ways that we think about energy aren't quite right. Refining petroleum uses about 6% of total energy in the country, but isn't considered when we think about fuel economy in cars. "If you think about it, every car you think is getting 40 miles a gallon is actually getting 30 miles a gallon, and maybe worse," Griffith said. Mining oil and gas uses even more energy.
The flowchart makes it clear that a huge amount of energy goes into infrastructure. "I think if you did a full accounting of this—what individual purchasing decisions do you make, what can you influence—I think you only influence about 40% of this," Griffith said. "To really solve this, we have to solve a lot of things at a deep infrastructure level. You need to be civically engaged."
Where do the oil sands fit in a low-carbon future?
JEFF RUBIN
Contributed to The Globe and Mail
Last updated Monday, Mar. 14, 2016 12:41PM EDT
Jeff Rubin is a senior fellow at the Centre for International Governance Innovation (CIGI) and the author of The Carbon Bubble: What Happens to Us When It Bursts.
Oil sands producers may have collectively breathed a sigh of relief on Prime Minister Justin Trudeau’s recent failure to get the premiers signing on to a national price for carbon emissions. However, domestic measures to reduce carbon emissions are the least of oil sands producers’ concerns when it comes to how actions to mitigate climate change will challenge their industry’s survival.
Efforts to abate the less than 2 per cent of global carbon emissions that originate from Canada are not what threatens the industry’s future in an age of profound and rapid climate change. Whether Canada lives up to its emission reduction pledges will have a negligible impact on world oil demand and global carbon emissions. The real threat that climate change poses to high-cost oil sources like Alberta’s oil sands is the actions taken to curtail the 98 per cent of global emissions that emanate from outside of Canada.
More than 190 countries committed to global emission reduction recently in Paris at the 21st session of the Conference of the Parties to the United Nations Framework Convention on Climate Change (COP21), and if we take them at their word, the future for high-cost oil is even bleaker than in today’s glutted oil market.
The international commitment to hold the increase in average global temperatures to less than a 2-degrees-Celsius rise requires the destruction of billions of barrels of future oil demand through either carbon taxes or through support given to renewable energy.
According to the International Energy Agency, holding atmospheric carbon to 450 parts per million and global temperature to an average increase of 2 degrees Celsius requires world oil demand to peak by 2020, and fall by more than 20 million barrels per day (bpd) over the next two decades. If the goal is to hold average global temperature to less than a 2-degree increase, as endorsed at COP21, even greater reductions in world oil consumption will be required.
Where will the oil sands fit in that world? It is the sector’s huge production costs, not its emission profile, that poses the greatest threat. As one of the most expensive sources of oil in the world, no improvement in oil sands emissions is going to safeguard production levels in a decarbonizing global economy that will be consuming less and less oil over time.
As is the case in clearing today’s glutted oil market, the cuts in future global oil consumption mandated by the need to mitigate climate change aren’t going to come from low-cost producers like OPEC. They are instead going to come from high-cost producers. U.S. shale production has already been cut back by more than 600,000 barrels a day over the last year and the U.S. Energy Information Administration is projecting more than a 500,000 bpd cut this year. It’s only a matter of time before more production from the oil sands, which has an even higher cost, is forced to shut in as well.
Instead of pursuing new pipelines to tidewater and global markets when even world prices no longer justify production costs, the industry needs to shift its focus to generating greater value added from what in all likelihood will soon be significantly lower levels of production. Considering that half of the nearly 2.5 million barrels of bitumen extracted every day isn’t even upgraded into crude oil, let alone value-added refined products such as gasoline or diesel, there is certainly lots of room to climb up the value-added ladder.
Whether the industry will do so is unclear. However, with Western Canadian Select, the benchmark price for oil sands fuel, trading as much as 50 per cent below world oil prices, the cheap feedstock price provided by Alberta bitumen potentially offers some of the most attractive refinery spreads anywhere in the world.
A contracting oil sands industry not only requires a change in economic strategy but in environmental policy as well. While production shut-ins will leave a fainter carbon trail in the atmosphere, they could soon leave a much larger footprint on the ground as oil sands mines are forced to close well before their economic lifetimes are over. Will taxpayers ultimately have to pick up the costs for decommissioning oil sands mines, and the reclamation of millions of metres of toxic tailing waste?
Over the past decade, Canadian economic and environmental policies have been designed to fast-track what at the time was seen to be the country’s principal engine of growth.
But as high-cost production from the oil sands becomes increasingly untenable in the emission-constrained global economy around the corner, both policies need to be rethought to meet the challenges posed by a sunset industry.